China Energy Storage Network News: Summary
Residential battery energy storage systems are not only an attractive option for consumers to save costs, but also by maximizing the use of renewable energy. Although the high cost of batteries has always been a major factor limiting the growth of energy storage deployments, the rapid growth of the electric vehicle (EV) market size will continue to drive down the price of lithium-ion batteries, which will further incentivize the rapid development of the battery market.
0 Preamble
Although residential users know little about the safety incidents that occur in battery energy storage systems, there are still security risks in using battery energy storage systems in residential environments. In response to this issue, this report is subject to an extensive study of the potential failure mechanisms of residential electric energy storage systems in the UK, the hazards associated with failures, risk mitigation, and existing safety standards and standards currently being developed. The report found that battery storage manufacturers and installers follow best industry practices and standards to significantly reduce the risk of residential battery energy storage systems in their applications.
Part of the assessment report involves working with stakeholders in order to better understand their concerns, gather their suggestions for improvement, and address the issues they face. An important point is that while the battery energy storage industry is still in its early stages, it is time to consider all the potential safety scenarios and how to properly operate battery energy storage systems, and the relevant safety standards and guidelines need to be developed, as battery energy storage systems will be widely deployed and gradually become part of people's daily lives. Therefore, establishing technically sound and meaningful safety standards is critical to the success of battery energy storage systems.
According to some recent studies, the main fire characteristics of lithium-ion batteries can be summarized into the following three risk categories:
When the battery fails and the heat runs out and spreads, too much heat is generated inside the battery pack, which highlights the need for a battery pack isolation design to minimize the risk of spread and limit the spread of fire between batteries/modules. Early detection and cooling methods as individual batteries begin to fail are critical to avoiding thermal runaway in battery energy storage systems.
Thermal runaway failure of a battery or battery pack can produce a large amount of toxic gas, which is caused by a rapid increase in pressure and the release of the exhaust port when the battery heats up. In the design and deployment of battery energy storage systems, the management and emission of gases generated by batteries must be considered.
When designing a ventilation system, special consideration needs to be given to how to deal with toxic gases generated by battery fires.
From battery cells to system design and system installation, there are many possibilities for risk mitigation at all levels. Many of the mitigation risks and requirements are included in existing or are being developed. Here are some considerations for risk mitigation:
Battery management systems (BMS) play a central role in keeping batteries within the operating window of voltage, current, and temperature. Safety standards for battery energy storage systems have specific requirements and tests applicable to battery management systems (BMS).
Although battery cell failures are rare, they do occur. For a well-structured 18650 battery, the failure rate for internal events is estimated at one in ten million (0.1ppm). This means that one battery in a 10,000-cell energy storage system fails (assuming a 5kWh battery storage system uses 500 18650 batteries). This is not to say that 1 in every 10,000 battery energy storage systems must fail, and there is a great risk of fire. The correct design and construction of a battery energy storage system should prevent the spread of battery failure. Because a single battery failure should be manageable.
If the system is well designed, the spread of thermal runaway events caused by a single battery should be considered. This is important for mitigating the risks to battery energy storage systems and will have a significant impact on their overall risk assessment. Controlling a single battery fault within a battery pack can reduce the risk of battery storage system failure and residential fire. Standards applicable to residential lithium-ion battery energy storage systems, such as BSEN62619 and IEC62933-5-2, include an assessment of the spread of battery failure.
The safety requirements of the Battery Energy Storage System in the United Kingdom can be divided into electrical installation requirements, power grid connection requirements, product safety regulatory requirements, and dangerous goods regulatory requirements. Its product safety involves several types of safety standards, such as electric energy storage systems, lithium-ion batteries, control and battery management systems, power electronic converter systems and inverters, and electromagnetic compatibility (EMC).
Some standards for lithium-ion battery energy storage systems are currently being developed or have recently been published. The recently released first edition of IEC62933-5-2 covers the security of residential battery energy storage systems. The upcoming version of the IEC62933-5-2 standard is likely to require lithium-ion battery energy storage systems to comply with standards such as BSEN62619, IEC62485-5 (under development) and IEC63056. If these standards are developed and published for the purpose of serving as harmonized standards under the Low Voltage Directive or the General Product Safety Directive, or cited in other standards or regulations such as BS7671, it will contribute to the process of clearly indicating compliance with the regulations. Current battery energy storage systems are typically only tested according to the BSEN62619 standard.
1. Introduction
Residential battery energy storage systems are not only an attractive option for consumers to save costs, but also by maximizing the use of renewable energy. This report reviews residential battery storage technologies and their applications to understand what further steps may need to be taken to mitigate risk. The focus is on lithium-ion battery technology, as it now dominates the new design of battery energy storage systems.
The study describes the operation and maintenance of battery energy storage systems, market conditions, and a review of released battery energy storage system fire data. Mitigations such as how lithium-ion batteries fail and best practices in battery energy storage design and installation with low risk of failure or impact are also elaborated.
Fortunately, there are few fire records of lithium-ion battery energy storage systems in the UK, so the report includes experiences and lessons learned from how to handle lithium-ion battery energy storage system fires in applications, as well as the causes and effects of thermal runaway, rapid increases in internal pressures, and the production of toxic gases.
2. Battery energy storage system
2.1, the design of battery energy storage system
Battery energy storage systems typically consist of the following parts: battery subsystem, energy storage system housing, power conversion subsystem, control subsystem, auxiliary subsystem, and terminal blocks (as shown in Figure 1).
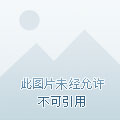
Figure 1 Simplified schematic diagram of the internal components of a battery energy storage system
2.2. Power conversion subsystem
The Power Conversion Subsystem (PCS) plays a key role in the transfer of energy to and from the power supply. It includes an inverter/charger and is usually designed in both directions, but may sometimes be a separate component. When charging a battery, it draws current from an AC power source (connection point) and converts it to direct current. When power is output to the grid or locally "self-powered," the inverter converts the DC power provided by the battery subsystem into AC power.
Battery energy storage currently on the market is usually one of the following three types:
Use an "off-the-shelf" bidirectional inverter.
Use proprietaryly designed inverters or retrofitted commercial products.
Bidirectional inverters, supplied only as "energy storage systems", require the installer, system integrator or end user to provide a suitable power converter.
The charger/inverter can separately manage the power input or output of the energy storage system, or it can integrate other local renewable energy sources, most commonly solar power generation systems.
2.3. Auxiliary subsystem
Auxiliary subsystems include all the necessary equipment designed to perform auxiliary functions of battery energy storage systems (e.g. heating, ventilation, fire protection, air conditioning systems).
2.4. Control subsystem
The control subsystem is used to monitor and control the battery energy storage system. It may include communication subsystems, protection subsystems, and management subsystems.
2.5. Battery subsystem
The battery subsystem consists of a battery management system (BMS) and batteries divided into multiple modules.
In order to operate safely, the battery needs to be kept within its voltage, temperature and current ratings, so a battery management system (BMS) is required to manage it. For larger battery energy storage systems, a separate battery management system (BMS) is often used to operate at different levels. A battery management system (BMS) is a critical part of a security system in a battery energy storage system, along with hardware protection.
There are many factors that must be considered when designing a safe battery subsystem. The design and engineering of battery management systems (BMS) is a critical issue for safety. Battery management systems (BMS) are at the heart of safe battery operation. If properly designed, a battery management system (BMS) will detect potential problems early and take action to mitigate the potential consequences.
3. Battery technology
Table 1 shows the characteristics of the most commonly used lead-acid and lithium-ion batteries in today's battery energy storage systems.
Table 1 Characteristics of lead-acid batteries and lithium-ion batteries
The battery technology used in battery energy storage systems may vary, but most of the batteries currently on the market for building battery energy storage systems are lithium-ion batteries. However, valve-controlled lead-acid batteries have traditionally dominated and are still used in many installed battery energy storage systems. For this application, the main disadvantage of lead-acid batteries is their limited life during charging/deep discharge. As a result, they are not typically used in residential battery energy storage systems. Therefore, the report does not introduce lead-acid batteries further.
3.1, lithium-ion battery
Lithium-ion batteries are made up of a series of different battery materials and components, the main reaction of which is the transmission and embedding of lithium ions into the cathode and anode respectively. The most common anode material is graphite, but there are also alternatives to hard carbon and titanate. Hard carbon and titanates provide better power capacity and cycle life, but the energy density is not high. While battery cathode materials are usually metal oxides, some of the most common cathode types are NMC (nickel lithide manganese cobalt oxide), NCA (nickel lithide cobalt aluminum oxide), LCO (lithium cobalt oxide) and LFP (lithium iron phosphate). They all have different characteristics, but lithium iron phosphate batteries (LPPs) have lower voltages and therefore have lower energy densities than other batteries.
The anode and cathode are separated by porous separators, which typically consist of a multilayer of polymer. Electrodes and diaphragms are soaked in an electrolyte, and the electrolyte between the electrodes can transport lithium ions. The electrolyte consists of an organic solvent dissolved in lithium salts (LiPF6).
3.1.1, lithium-ion batteries
Today there are three different shapes of batteries: cylindrical, soft pack and prismatic. Electrodes can be stacked or wound. The battery is sealed and should not be vented under normal operation.
Different form factors for lithium-ion batteries
Batteries are connected in series and parallel to increase voltage and capacity, respectively. For larger battery packs, the batteries are typically grouped into subgroups called modules, which can then be connected in series or in parallel to form a complete battery pack.
The production and construction of batteries can be quite complex. While the application of existing battery and system standards involves performance and safety, although they are constantly reviewed and improved, they do not completely address all the complexities involved in battery design and manufacturing, so there are occasional battery failures. Modest changes in chemistry or structure can have serious consequences. From a safety point of view, it should be remembered that there are billions of batteries on the market today, and from this point of view, the accident rate is actually very low, about one part per million or possibly as low as one in ten million.
4. Review of the UK energy storage market
Battery energy storage systems deployed in the UK market are usually from global suppliers and UK manufacturers, and the number of suppliers is expected to increase in the next few years. The price of battery energy storage systems can vary greatly. According to a survey report, battery prices range from £500 to £10,000 and do not include installation costs. The cost of a battery energy storage system (excluding installation costs) averages around £1,000/kWh. Depending on the complexity of the energy storage system, its installation costs can range from £500 to £2,000 or more. Some battery energy storage systems also use inverters, which could cost around £1,000.
4.1. Installation example of battery energy storage system
Here are two examples of installing a residential battery energy storage system. It should be noted that neither of these battery energy storage systems is installed in the living area.
Varita-Storage 'Pulse' battery energy storage system installed in the garage
Tesla Powerwall battery energy storage system installed on outdoor walls
4.2, the British market battery energy storage system example
The products that can be found on the UK market are shown in Table 2. The table summarizes the information on each battery storage system manufacturer's network. The energy storage capacities of these residential electric energy storage systems range from between 2.5 and 25.2kWh. The nominal voltage of the battery module is generally around 50V, but some battery energy storage systems have a higher nominal voltage. Although it is not possible to find the rated capacity of each battery module for each energy storage system, however, it ranges from between 1kWh and 6.3kWh. The battery used in the battery energy storage system is usually a lithium nickel manganese cobalt oxide (NMC) battery or a lithium iron phosphate (LiFePO4) battery.
These battery products all carry the CE mark. A common battery safety standard for battery module testing is IEC62619. For inverters, IEC62109-1 and IEC62109-2 are commonly used safety standards. Many residential battery energy storage systems are also certified for the North American market according to standards such as UL1973 (battery module) and UL1741 (inverter). Specifically, for the UK market, the inverter also needs to comply with engineering recommendations G.83/2 (G98) and G.59/3 (G99). The applicable standards for domestic BESS are summarized in Section 7 and Appendix 1.
Table 2 Battery energy storage system products used in the United Kingdom
Author: Compiled by Liu Boxun Source: China Energy Storage Network