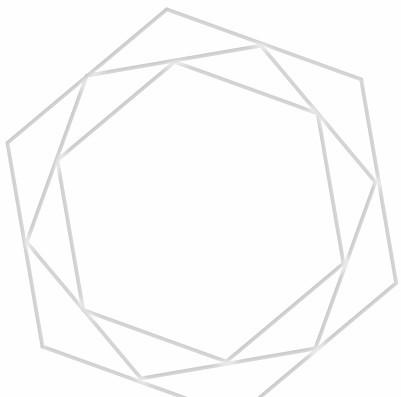
Follow the voice of the storm
Elevate your thinking
Guide
Extremely dense metal hydrogen under ultra-high pressure is considered to be the highest energy-containing material after nuclear energy materials.
This article is excerpted from Engineering, No. 8, 2021, journal of the Chinese Academy of Engineering
Author: Mao Heguang, Ji Cheng, Li Bing, Liu Gang, Eugene Gregoryanz
来源:Extreme Energetic Materials at Ultrahigh Pressures[J]. Engineering,2020,6(9):976-980.
Editor's Note
Energetic materials store large amounts of chemical energy that are rapidly released under thermal or force stimuli. Extremely energetic materials generally refer to single-bond polymeric nitrogen and single-atom metal hydrogen containing extremely high energy densities. At present, the research on extremely energetic materials under ultra-high pressure is still in the exploratory stage. Although extreme energy-containing materials require ultra-high pressure of several hundred gigapas per square meter to synthesize and are difficult to apply directly, studying the stability, metastability and basic properties of these materials can provide valuable information for finding extremely energetic materials through other synthesis paths.
Mao Heguang, a foreign academician of the Chinese Academy of Sciences, and his scientific research team published "Extreme Energetic Materials under Ultra-High Pressure" in the 6th issue of Engineering, the journal of the Chinese Academy of Engineering, in 2020, introducing the ultra-high pressure research of two extreme energetic materials of elemental nitrogen and elemental hydrogen, and pointing out the future development prospects and cutting-edge problems that need to be solved. The article points out that among the many kinds of polymeric nitrogen that have been discovered, cubic deflection structure polymeric nitrogen and black phosphorus structure polymeric nitrogen are two particularly interesting polymeric nitrogen, and their lagging conservation phenomenon provides a prospect for further exploration of nitrogen applications; metal hydrogen is considered to be the highest energy-containing material second only to nuclear energy materials, mainly focusing on the wonderful evolution process of the crystal structure and electronic structure of molecular hydrogen under pressure, that is, from low-density ultra-wide energy band insulators, to narrow energy band semiconductors, to high-density molecular metals and atomic metals. Finally, to a new physical state like never before.
I. Introduction
Stress changes all substances and has an impact on the scientific study of all substances. The current pressure of diamond anvil (DAC) can reach a few hundred gigapas, which directly changes the crystalline structure, electronic structure and chemical bonds of condensed matter, and can be used as an ideal tool for finding extremely optimized energy-containing materials such as the most efficient fuels or the highest-energy explosives. However, useful energetic materials must be able to be preserved in a conventional environment in order to be practical. Therefore, the research of exploring energetic materials under extreme pressure should be divided into two steps: one is pure curiosity-driven scientific research, casting a wide net to discover and explore many novel materials and their properties under high pressure; the other is scientific research driven by the application of new materials, using new knowledge obtained under high pressure to directly recover or use chemical methods to synthesize the novel materials found. For example, superhard diamond is initially synthesized under high pressure and then successfully recovered under atmospheric pressure after pressure release to metastable diamond for use in industrial abrasives. Later, using the knowledge of the diamond sp3 chemical bond, the gem-grade diamond can also be grown by chemical vapor deposition (CVD) under substable conditions of zero pressure.
The research on extreme energy-containing materials under ultra-high pressure is still in the exploratory stage. In this article, we discuss the ultra-high pressure study of two extreme energy-containing materials, elemental nitrogen and elemental hydrogen. N2 and H2 start with gases, which are the most easily compressed materials, which makes in-situ testing at hundreds of gigapascals extremely challenging. N2 and H2 each have their own unique and enticing scientific significance, as well as the same technical challenges. For example, how do you load a thin gas into a DAC and press it into a solid thousand times denser? How can Raman and infrared (IR) spectroscopy be used to track the evolution of their molecular bonds under ultra-high pressure? How do X-ray diffraction (XRD) be used to determine their crystal structure and equation of state? How can electronic transport measurements be used to account for changes in their electronic structure? Relatively speaking, nitrogen research is easier than hydrogen and often serves as a precursor to the study of hydrogen. For example, an open DAC can be pressed and loaded with a nitrogen sample directly in liquid nitrogen, which requires complex compression and safety protection systems. The intensity of X-ray scattering is proportional to the square of the atomic number, so that the XRD signal of the hydrogen atom is 1/49 of that of the nitrogen atom. Polymeric nitrogen can form at pressures below 150 GPa, while metallic hydrogen requires pressures above 500 GPa to form and enter areas where DAC experiments are difficult to replicate and pressures are poorly calibrated. In short, the high-risk-high-reward scientific pursuit of nitrogen and hydrogen is becoming a key driver of ultra-high pressure detection technology innovation.
Second, nitrogen under ultra-high pressure
(a) Three-bond molecular nitrogen
Much of the progress of explosive materials in modern times is based on the development of high-nitrogen compounds. The ultimate extreme material, of course, is single-bond elemental pure nitrogen. Under normal conditions, pure nitrogen is composed of three-bonded N2(N≡N) diatomic molecules that solidify at low temperatures or pressures into crystals of nitrogen molecules linked by van der Waals forces. Theory predicts that under sufficient high pressure, nitrogen molecules will break the triple bond, forming a three-dimensional structure of polymeric nitrogen between nitrogen atoms that binds to a single bond. Due to the very high binding energy (4.94 eV per atom) contained in the three bonds of nitrogen, polymeric nitrogen that changes from three bonds to single bonds stores great energy. The explosion heat per unit mass of polymeric nitrogen is 2.9 times that of pentaerythrityl tetranitrate (PETN, C5H8N4O12), 6.7 times that of trinitrotoluene [TNT, C6H2CH3(NO2)3], and 10.7 times that of lead azide [LA, Pb(N3)2]. The search for single-bond nitrogen began in the 1980s, prompting the development of high-pressure DAC technology, which in turn used XRD to probe crystal structures, and The use of Raman and IR vibration spectroscopy to explore changes in nitrogen bonds under pressure. Related studies in the 20th century have revealed complex phase diagrams of nitrogen at 120 GPa and different temperatures, including at least 7 stable phases (α, β, γ, δ, ε, lambda, ζ). Each phase above 120 GPa has its own crystalline structure and vibration spectrum, but its basic building block is still N2 (Figure 1).
Fig. 1 Phase synthesis of nitrogen. CG: cubic deflection structural phase; BP: black phosphorus structural phase; LP: layered structure polymeric nitrogen. The image has been approved by Ji, etc
(2) Single-bond polymerization of nitrogen
At temperatures above 300 K and pressures above 150 GPa, nitrogen becomes an opaque, conductive, non-molecular amorphous state (η nitrogen). N2 molecular vibration Raman and IR peaks disappear, while new wide peaks appear. The disappearance of the XRD Bragg peak of nitrogen indicates that it has become a non-molecular amorphous polymeric nitrogen. The amorphous η phase caused by pressure is metastable, in which the N2 triple bond is broken and becomes a single bond of the polymeric nitrogen η phase.
The process of phase transition to η phase exhibits significant lag. At a temperature of 300 K, η phase polymeric nitrogen requires more than 150 GPa of pressure to synthesize, but after synthesis, the pressure is released to 50 GPa, and the η phase can still be preserved. Since the reaction force decreases exponentially with the decrease of temperature, the low temperature is more conducive to lag. At a temperature of 100 K, polymeric nitrogen can even be stored at zero pressure. In general, the use of high-temperature accelerated reactions to synthesize the desired phase and the use of low-temperature inhibition reversal to preserve the metastable phase are effective strategies for synthesizing novelty materials.
(3) Cubic deflection structure and other single-bond nitrogen
Although the use of room temperature high pressure synthesis - low temperature atmospheric pressure recovery has successfully demonstrated how to bring the high pressure phase back to atmospheric pressure, we prefer to make the final product available for direct use at room temperature through high temperature and high pressure synthesis - normal temperature and atmospheric pressure recovery. Eremets et al. compressed nitrogen to 110 GPa in the DAC and heated it to 2000 K with a laser, and observed that the XRD spectrum of nitrogen exhibited the single-bond cubic deflection (CG) crystal structure predicted by earlier theory. Cubic deflected nitrogen (CG-N) has also been repeatedly verified in subsequent high temperature and high pressure experiments. Then the theoretical calculations used the newly invented structural evolution search method to predict many new structures of high-pressure polymeric nitrogen. In the laser-heated DAC experiment, Raman spectroscopy was used for identification, and single-bond crystalline polymeric nitrogen with a layered crystalline (LP) structure was found at 120 to 180 GPa, and polymeric nitrogen with hexagonal layered crystalline (HLP) was found at a pressure of more than 240 GPa. These steady- and metastable polymeric nitrogens coexist in the same temperature and pressure intervals, indicating that their generative free energy is almost the same, while the energy barrier between them is very high. This provides favorable conditions for the recovery of novel and useful materials outside the thermodynamic stabilization zone.
(4) Nitrogen of the structure of black phosphorus
By analogy with phosphorus under normal conditions, we can help us understand nitrogen. Elemental phosphorus has 4 isotropics: white phosphorus, red phosphorus, purple phosphorus, and black phosphorus (BP). These 4 allotropes have very different physical properties and uses, and can all be stored indefinitely at room temperature and pressure. Both nitrogen and phosphorus belong to the V group of elements in the periodic table, located in the first and second cycles, respectively. The general law of high-pressure science is: "Pressurization makes an element more like the elements of the next cycle of the congener." "Of particular interest are the single-bond BP allotropes, which are representative of two-dimensional (2D) materials specific to group V elements, whose layers show strong anisotropy (i.e., wrinkles in one direction and smoothness in the other). The BP structure is present in all group V elements except nitrogen, including phosphorus (P), arsenic (As), antimony (Sb), and bismuth (Bi). Exhibiting many excellent electronic, mechanical, optical and transport properties, they are considered potential to surpass the flat two-dimensional layer of group IV elements led by graphene and develop into the next generation of two-dimensional materials. However , among the elements of known BP structure , nitrogen , led by group V elements , is lacking. The thermal letter calculation also shows that nitrogen (BP-N) of the black phosphorus structure is not steady state at high pressure and low temperature, but this does not mean that BP-N cannot be synthesized at high temperature and pressure and stored in a metastable state at low temperature. We found BP-N at high temperature and pressure and found that this temperature and pressure zone overlaps considerably with the pressure and temperature zone where CG-N and layered polymeric nitrogen (LP-N) can be synthesized and preserved (Figure 1). XRD and Raman spectroscopy showed that BP-N is a single-bonded nitrogen with a folded two-dimensional structure, so it is not only an extremely energetic material, but also a new generation of two-dimensional materials. Like CG-N, BP-N has considerable hysteresis and can be stored in a metastable state over a wide high-pressure area after synthesis. It becomes N2 only at temperatures of 300 K and pressures below 48 GPa.
(5) Prospects of polymeric nitrogen as an energetic material
The lag in the performance of crystalline and amorphous polymeric nitrogen reflects the huge energy barrier between single- and triple-bonded nitrogen, which is conducive to the recovery of newly discovered high-pressure phases as material applications. Recently, CG-N has been successfully synthesized under atmospheric conditions, which uses radio waves to promote the production of plasma from a mixture of nitrogen and argon, and reacts with β-sodium azide on multi-walled carbon nanotubes, further increasing the possibility of energy-containing material applications. Doping is another strategy for designing synthetic materials by extending metastability. Nitrogen is at a favorable intersection on the periodic table, with neighboring co-periodic elements boron and carbon and congenerial next-cycle element phosphorus both having strong metastables and multiple allotropes. Nitrogen is easy to form compounds under high pressure, and polymeric nitrogen can also be synthesized by various dopings. In the future, the application of oriented polymeric nitrogen should consider the design and synthesis of BP-N or other single-bond polymeric nitrogen by chemical pathways under near-normal conditions, and consider the use of nearby elements as a substrate for chemical doping or epitaxial growth to stabilize polymeric nitrogen.
Third, hydrogen under ultra-high pressure
(1) Hydrogen as an extremely energetic material
Low-density gaseous and liquid hydrogen has long been used as an important energy material in large quantities, and its wide range of uses involve rocket propellants and automotive fuel cells. The extremely dense metal hydrogen under ultra-high pressure is considered to be the highest energy-containing material after nuclear energy materials. Because of its extremely high hydrogen density, it can even be used as the most efficient feedstock for nuclear fusion. Metallic hydrogen has the potential to exceed room temperature high-temperature superconducting materials, as evidenced by the extremely high superconducting transition temperatures (Tc) of lanthanum (La) and yttrium(Y) rich in hydrogenides. However, it is still impractical to treat metallic hydrogen as a truly usable material. First, the metal hydrogen produced using existing technology is only the order of Pic and is still insignificant even if used as a feedstock for nuclear fusion. Second, and more importantly, hydrogen transitions instantaneously without lag, and theoretical calculations predict that even if metal hydrogen is synthesized, the life of metastable metal hydrogen placed at atmospheric pressure is not as good as picoseconds. Finally, although the heat of hydrogen production in metals is 76 times that of TNT at a pressure of 500 GPa, this extra energy comes from the massive elastic compressibility of hydrogen and cannot be preserved. All in all, the study of hydrogen as a material application under ultra-high pressure is still in its infancy, while curiosity-driven basic science and a series of unexpected inventions are waiting to be explored.
(2) The pursuit of metallic hydrogen
Ginzburg said it well that metallic hydrogen is a key problem in physics and astrophysics in the 21st century. It is a multifaceted question that has different meanings and appeals to many different professions. For astrophysicists, metallic hydrogen is the most abundant matter in the universe, hidden deep inside celestial bodies under extreme high pressure (Figure 2, liquid metallic hydrogen). For condensed matter physicists, it may be the highest temperature superconductor and the highest energy-containing material. For theoretical physicists, it is a previously unknown new physical state, which at absolute zero may be a binary (electron and proton) superfluid or superconductor. For experimentalists, it is the Holy Grail of ultra-high-pressure physics, hanging outside the limits of the pressure and detection techniques that can be achieved, inducing us to go further. Scientists' interest and understanding of metallic hydrogen is up to date and in line with contemporary hotspots. In 1935, when the electronic band structure became the cornerstone of solid-state physics, single-electron metallic hydrogen was predicted to be representative of the purest free electron alkali metal band. In the 1960s, when the electronic phonon-coupled Bardeen-Cooper-Schlieffer (BCS) microscopic theory of superconducting states was in vogue, metallic hydrogens with ultra-high phonon frequencies were predicted to be ultra-high temperature superconductors. In the early 20th century, when the Bose-Einstein condensate took center stage in physics, the intense quantum effects of electrons and protons of metallic hydrogen were concerned, thought to create binary superfluids. Recently, two-dimensional layered graphene has emerged as an emerging material, and the structure of high-pressure hydrogen is also speculated to be composed of two structures: graphene and bromine molecules (Br2). Now it seems that metallic hydrogen is more of an outdated term. It was a novelty and surprise to recognize in 1935 that pressure could cause hydrogen metallization, and that metallization is now observed in thousands of substances, which is expected to be universal. Therefore, the real meaning of pursuing metallic hydrogen should be to understand the colorful evolution of hydrogen under pressure, that is, from the crystal of a diatomic molecule to the final state of the atom (which may be composed of binary superfluids or other unexpected new physical states). The process has a series of continuous or transition structural and electronic variations, from insulators with low-density ultra-wide energy bands to semiconductors with narrow energy bands, to molecular metals with high density and no energy gaps, and finally to alkali metals in atomic states or other novel unknowns.
(3) The frontier of today's experiments
The metallization of solid and liquid hydrogen has been observed in many static and dynamic high pressure experiments, but our task is far from complete. The key problem is the lack of ability to observe fundamental physics phenomena under the pressure limits of daCs, so that conclusions are often controversial. Even though the hydrogen electron gap was observed in experiments to narrow step by step with pressurization until it disappeared, which is an important milestone in technological progress, it has limited gold content in scientific innovation. The real mission of metallic hydrogen is to unearth unexpectedly rich new physics phenomena by exploring extreme variations of hydrogen in the vast compression range. The prerequisite is to be able to monitor the fundamental properties of hydrogen samples as they change, especially the two pillars of condensed matter physics: crystalline structure and electronic band structure. The most common detection methods are optical Raman and IR spectroscopy, which can be used to study the vibrational properties of H2 molecules until the highest pressure hydrogen sample becomes opaque. These spectroscopic techniques are used to map the phases of hydrogen and to define the molten and five crystalline phases from low pressure to 400 GPa: I, II, III, IV, V (Figure 2). These vibrational spectra primarily observe individual molecules and provide only indirect information about the crystal and electronic structure of the condensed matter core.
Fig. 2 Phase diagram of hydrogen. The images have been approved by Gregoryanz et al
We have developed a range of X-ray probe techniques using high-brightness, high-energy synchrotron radiation sources to study the fundamental physical properties of DAC high-pressure hydrogen. Using the optimized high-pressure XRD technology to track the crystal structure of hydrogen with a pressure of up to 245 GPa, it is found that the iiith and IV phases of hydrogen always maintain a hexagonal compact (HCP) structure, but the lattice parameters are significantly lower than c/a, indicating that there may be an electron topological phase transition. This represents the frontier limit of current exploration of hydrogen crystal structure. As for the electronic band structure of hydrogen, in addition to the indirect data of optics, it has not yet been able to be directly tested under high pressure.
(4) Break through the frontier
With the scientific goal of discovering the rich new physics of hydrogen's long and tortuous path from molecular insulators to atomic metals, we still have a big knowledge gap to fill. Cutting-edge questions include: What is the structure of phase V before a molecule breaks down into atoms? What is the electronic band structure of these 5 phases? How do molecules interact with each other in crystals causing phase transitions and molecular decomposition? The answer lies in technological innovations, especially the ever-changing capabilities of synchrotron radiation light sources. Continuing to optimize the high-voltage XRD technology should be able to advance the transformation of the crystal structure from phase IV to phase V to the limit of molecular hydrogen. In addition, we can also develop a high-pressure medium energy resolution (eV) X-ray inelastic probe technology similar to the successful study of high-pressure helium electron band structure, to create the first research on the structure of hydrogen electron bands.
The changes in the process of hydrogen metallization are mainly caused by the interaction between hydrogen molecules caused by pressure. The optical Raman spectroscopy of the oscillator measures the vibration of the same phase between hydrogen molecules, while the IR spectrum measures the vibration of the staggered phase, and the difference between the two is the interaction between the molecules. However, the low-energy optical range momentum is very small, and Raman and IR information is limited to the center of Brillouin. This problem can be solved by using high-energy synchrotron radiation X-rays to cover the entire Brillouin area. The development of inelastic probes with high pressure high energy resolution (meV) can measure the changes of hydrogen vibrators in the main branches of all Brillouin regions, thereby exploring the role of hydrogen molecules in the three-dimensional lattice. This will be the main breakthrough direction for the study of ultra-high density hydrogen molecular systems.
To go deeper into the region of atomic hydrogen, we face entirely new challenges: without molecular bonds and lattice, Raman spectroscopy and XRD are powerless. The most critical question is, how can we seal the atomic hydrogen of the superfluid with DAC? What evidence is used to detect superfluids, binary superfluids, or other novel physical states? Solving these problems is the real meaning of the experimental exploration of metal hydrogen.
Note: The content of this article is slightly adjusted, if necessary, you can view the original text.
Adapted original text:
Ho-Kwang Mao, Cheng Ji, Bing Li, Gang Liu, Eugene Gregoryanz.Extreme Energetic Materials at Ultrahigh Pressures[J]. Engineering,2020,6(9):976-980.
About the author
Mao Heguang is a geophysicist, an academician of the National Academy of Sciences and a foreign academician of the Chinese Academy of Sciences.
For the first time, the problem of generating and calibrating millions of atmospheres was solved, and the highest international static pressure of 5.5 million atmospheres was obtained. A variety of experimental methods and experimental systems for in situ measurement of the structure and properties of matter under high pressure have been created, and a lot of pioneering work has been done in the fields of high pressure physics, high pressure chemistry, high pressure materials science, and earth and planetary interior physics.