1
Birth in controversy
In the early 20th century, after Einstein published his theory of general relativity, there was a great debate in the scientific community about the state of the universe: whether the universe was static, as Einstein believed at the time, or dynamic, as predicted by the Russian physicist Alexander Friedman in 1922 based on the theory of general relativity. This debate about the basic state of the universe will be distinguished by experimental observations.
In 1929, the American astronomer Edwin Hubble published a major discovery based on a series of Extra-Galactic Nebulae observations[Note 1]: the apparent receding velocity of the nebula v and its distance from D were roughly proportional to each other [1] (Figure 1), i.e. v = H×D. This suggests that the farther away the nebula, the faster it regresses, which in turn indicates that the universe is expanding and that the universe is dynamic. Hubble's findings are thus recognized as the first observational evidence for the theory of the expansion of the universe. v = H×D is called Hubble's law, which is included in cosmological textbooks, and the scale factor H in it is called the Hubble constant, which represents the current rate of expansion of one unit distance in the universe.
The fact that the universe expanded undoubtedly opened up a new chapter in human understanding of the universe, and the big bang theory of the universe also developed in the following nearly a hundred years, and was confirmed by more observations. It should be noted that as early as 1927, two years before Hubble published his results, the Belgian priest and cosmologist Georges Lemaitre theoretically deduced in an article[2] that the receding velocity of stars was proportional to their distance, which became known as Hubble's law. Unfortunately, Lemaitre's 1927 article was published in French-language journals, and much of the English-speaking world failed to see his results in the first place. Although the article was translated into English in 1931 and published in the monthly journal of the Royal Astronomical Society,[3] it was two years after Hubble's article. Hubble's 1929 observations do not cite Lemaitre 1927 (or Friedman's 1922).[4] The English translation missed several key details in the French version, which may have contributed in part to Lemytre's less well-known results than Hubble's. In 2018, the International Astronomical Society (IAU) voted to change Hubble Law to Hubble-Lemaitre Law. So far, after nearly a century, Lemaitre's status has been somewhat certified. However, the IAU's motion itself has also been controversial. The evaluation of scientific contributions involves many complex social factors, and the debate is likely to continue.
Many major breakthroughs in the history of science are based on the long-term accumulation of the entire scientific community, forming the conditions and environment for breakthroughs, and then a small number of scientists or teams complete the work in the appropriate historical period. To use an inappropriate analogy, just as a group of painters collaborate to create a huge portrait, the person who completes the final finishing touch is important to the formation of the portrait, but the contributions of others are undeniable. Hubble's observations are no exception, and before Hubble's observations, the scientific community already had clear expectations of the expansion of the universe (e.g., Friedman 1922, Lemaitre 1927), and in this sense, they, and the entire community of scientists involved in this research, are admirable on the road to exploring the expansion of the universe.
Figure 1. The law reflecting the regression velocity of distant galaxies v proportional to their distance from Earth, v = H×D (left, from reference [1]), was voted by the International Astronomical Union in October 2018 to change the original name hubble law to hubble (center)–Lemaite (right) law, in recognition of Maillert's contribution to the discovery of this law.
2
Ignorant of the ever-changing infancy
The Hubble constant is a key fundamental constant in modern cosmological models: H = v/D, velocity divided by distance, which represents the current rate of expansion of the universe and is also directly related to the age of the universe (1/H is the time, which directly gives an order of magnitude estimate of the age of the universe). Therefore, since 1929, researchers have been working hard to continuously improve the measurement accuracy of H.
Measuring H seems so simple that schoolchildren can do it, we only need to measure the regressive velocity of a distant star relative to us v, and its distance from us D, we can directly use their ratio v/D to get H0. In fact, this is exactly what Hubble did in 1929, except that he used not just one, but 24 "extragalactic nebulae" to limit H to narrow the error. But the measurement of H proved challenging, and between 1929 and the end of the 20th century, astronomically accepted Hubble constant measurements varied dramatically and controversially. Mainly because we are very difficult to determine the distance D of distant stars.
Hubble estimated H=465±50 km/s/Mpc in 1929, and Lemaite's 1927 estimate was close within the margin of error. The age of the universe speculated from this value is about 2 billion years, and at that time, geologists estimated the age of the Earth to be about 3 billion years using the decay of radioactive isotopes in rocks, which is obviously unreasonable. The age of the universe is inversely proportional to H, which shows that the H value given by Hubble is too large. In 1952, the German astronomer Walter V. Walter Baade announced that the H he measured was about half of what Hubble had originally measured, and that the key to the change was the galaxy's distance measurement.
Hubble used its circumference relationship to determine the distance of galaxies by assuming that all Cepheids are classical,[Note 2] and Bud observed that cepheids also have two different types, classical and second,[7] and that the two cepheids follow different circumference relationships. With this correction, Bud lowered the value of H, thus solving the contradiction between the age of the universe and the age of the Earth. In the late 20th century, estimates of hubble's constant H were declining. The most famous event of this period was the long-standing debate between Gérard de Vaucouleurs and Allan Sandage, who believed that H would be about 100 km/s/Mpc, while the latter believed that H would be as low as about 50 km/s/Mpc.
3
Harmonious and beautiful development period
At the beginning of the 21st century, a reasonable and widely accepted H measurement finally appeared. In 2001, the HST key project team led by american astronomer Wendy L. Freedman estimated H=72±8 km/s/Mpc based on observations in the nearby universe (usually redshifts of less than 0.1 or 0.15) of Cepheids and Type Ia supernovae. This value is exactly between the results of Devogure and Sandage, which ends the debate between The Dvorgule and Sandage teams. Subsequently, observations from the early universe broadly supported Freeman's measurements in the nearby universe, further affirming the scientific community's confidence that H was about 70 km/s/Mpc.
For the early universe, the linear expansion behavior described by the Hubble-Lemay-specific law failed, and the cosmological distance depended on the cosmological model. The standard cosmological model based on the discovery of the accelerated expansion of the universe in 1998 [9,10] allows scientists to use distance-redshift data from high redshifts to determine the Hubble constant. The most distant signal that humans can observe today is radiation from the afterglow of the Big Bang , the cosmic microwave background (CMB) radiation. The CMB radiation we measured revealed the distribution of matter about 380,000 years after the birth of the universe, and this data contains a wealth of information that can be used to measure many cosmological parameters, including dark matter, dark energy, and hubble constant, provided that we assume a cosmological model. But from another point of view, measuring hubble constants with CMB data, from method to data, is completely independent of the measurements of the nearby universe, and it is ideal to be able to measure the same physical quantity with such independent experiments.
The most famous CMB observation satellite of the early 21st century was NASA's Wilkinson Microwave Anisotropy Probe (WMAP), launched in June 2001. WMAP published its first observations in 2003, followed by follow-up observations in 2007, 2009 and early 2013, and the project's estimates of H based on CMB observations and standard cosmological models are always substantially consistent with those given by nearby cosmic observatories. Such results have amazed many physicists: two completely independent sets of experiments, independent data, and the data itself span tens of billions of years of cosmic history, and the results are actually consistent. If this result is true, we have to marvel at the harmony of the universe (the standard cosmological model); we also have reason to admire humanity's great achievements in the precision and accuracy of scientific measurements. This period can be seen as a harmonious and beautiful development period of the Hubble constant (Figure 2).
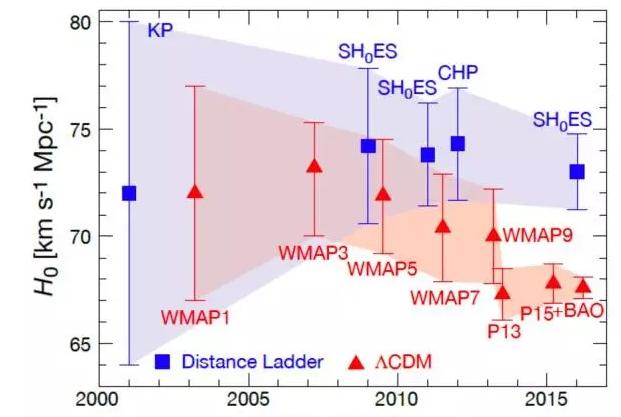
Figure 2: The harmonious and beautiful development period of the Hubble constant (2001-2012). The plot shows the measurement of H0 between 2001 and 2016; the horizontal axis is the year and the vertical axis is the measurement of H; where the blue point with error bar represents the H value given by the near neighbor cosmic observation, and the red point with error bar indicates the H value given by the high redshift observation. It can be seen that before the point of P13 (indicating the results of the Planck satellite published in 2013, which will be mentioned later), the blue and red areas are compatible with each other (image: References [12])
4
Contradictions abound in the present dynasty
In today's era of so-called "exact cosmology", the accuracy of the Measurement of the Hubble constant is once again highly controversial as the measurement accuracy of the measurement is improved step by step.
The event quickly sparked a heated debate in the astronomical world. In the years that followed, both project teams continued to review and update their respective measurements, and even check each other's mistakes and omissions, but the problems that emerged did not alleviate, but became more and more serious. The difference between the two sets of values has risen from the previous 3 standard deviations to more than 4 standard deviations today, one to the left, one to the right, and gradually drifting away (Figure 3). The latest analysis given in a preprint article [15] published by the Riess team in early last month (early December 2021) shows that the difference between the two sets of values has risen to 5 standard deviations. It can be seen that the difference between the two is an inevitable fact.
Figure 3. The years of frequent contradictions in the Hubble constant (2013-present). The figure on the left shows the H measurements published in recent years, where the point with the red error bar represents the H value given by the low redshift observation, and the blue point with the error bar indicates the H value given by the high redshift observation (image from the reference [16]). The distribution and trend of these two sets of values are easily reminiscent of the picture book cartoonist Jimmy's "Go Left and Right"
5
The possible outcome of the debate
Over the past few years, the scientific community's discussion and attention to the H problem has been in full swing, and papers have been published in this field almost every week, and authors often try to resolve, or claim to solve, this contradiction. But as of Christmas Eve 2021, this contradiction has not been accepted to be resolved. So what does it mean that the early cosmic data's definition of H is inconsistent with the direct measurements of the nearby universe? There are three rough possibilities:
First: The Planck data, or data from nearby cosmic measurements, and the process of their analysis may be problematic. This suspicion is probably difficult to completely rule out, because the observation and data analysis in both fields are extremely complex, and it is a team, not a single person, according to a certain process of analyzing the results obtained, this process can be 100% repeated in principle, but in fact no one has repeated 100%. Most retests always focus on the most likely problematic areas, with the examiner's prior judgment. If there are factors of error that we did not expect, it is difficult to investigate. In fact, the Planck team and the Riess team have tried several times to do in-depth investigation of their own analysis, and have repeatedly questioned each other's analysis, but the results have not found obvious problems. Therefore, the big problems in the data and data analysis process have not been found so far, and it is difficult to exclude 100%, and it is estimated that more in-depth investigation will continue.
Second: there is no problem with data analysis on both sides, it is the existing model of the universe that has problems, and our understanding of physics is problematic. This is possible, but this possibility is difficult to prove or falsify for a while. It is true that the standard cosmological model is a bridge connecting the early universe and the late universe, and the H obtained with CMB data is based on model assumptions, and those who question the standard cosmological model often propose to modify the expansion history of the universe, so that the distribution of matter in the early universe is in line with the CMB data, and the late expansion is slightly faster, so that it is possible to make the CMB data also consistent with the expansion rate directly measured by the late universe, such as modifying the gravitational model or proposing the early dark energy model, such models are not in the minority, Often by introducing additional degrees of freedom, new models can be adapted to the observational data within a particular parameter interval. However, there is currently no theory of modifying the Standard Model that has been widely accepted. Perhaps the development of physics has always been biased toward the beauty of simplicity and harmony, and the standard cosmological model based on general relativity, although there are still many unsolved mysteries, has successfully explained countless observations, and the physical community does not seem to have found enough reason to accept its revised version.
Third: we have not yet imagined other possibilities. In the history of the development of physics, controversy seems to be the norm; it means the existence of problems, and the development of physics often begins with the question of asking new questions. The more the so-called truth is argued, the more clearly debated it is likely to mean the next breakthrough in understanding. At the end of the 19th century, the physics community also believed that the classical theory of physics at that time had explained all physical phenomena, with only two exceptions, and the attention and discussion of those two exceptions later led directly to the discovery of quantum mechanics and general relativity, opening up a whole new dimension of human understanding of the world.
Today's debate about the Hubble constant itself illustrates the existence of this problem, and perhaps a rare opportunity. I believe that an open, transparent and continuous discussion of this issue will bring us a new understanding of the universe.
★ Note 1: At that time, Hubble and the astronomical community did not know that there were milky way-like "extragalactic galaxies" beyond the Milky Way, so Hubble called it an "extragalactic nebula".
★ Note 2 to entry: Cepheids have periodic variations in brightness, and their period of change is related to its brightness, the so-called "period-luminosity" relationship, or week-light relationship, so we only need to measure the period of change of Cepheids to deduce its absolute brightness, and then know the distance between the galaxy in which it is located and us.
bibliography:
[1] Hubble, E., (1929) "A relation between distance and radial velocity among extra-galactic nebulae". Proceedings of the National Academy of Sciences. 15 (3): 168–73.
[2] Lema tre, G., (1927) "A homogeneous Universe of constant mass and increasing radius accounting for the radial velocity of extra-galactic nebulae", Annales de la Société Scientifique de Bruxelles, A47, p. 49-59
[3] Lema tre, G., (1931) “Expansion of the universe, A homogeneous universe of constant mass and increasing radius accounting for the radial velocity of extra-galactic nebulae”, MNRAS, 91, 483L
[4] Friedmann, A. (1922), "über die Krümmung des Raumes." Zeitschrift für Physik, 10, 377-386.
[5] Livio, M., (2011) “Lost in translation: Mystery of the missing text solved”, Nature, 479, 171
[6] Kragh, H. (2018) “Hubble Law or Hubble-Lema tre Law? The IAU Resolution”, arXiv:1809.02557
[7] Baade, W. (1956) “The period-luminosity relation of the Cepheids”. Proceedings of the National Academy of Sciences. 68, 5-16
[8] Freedman, W. L., et al. (2001). "Final results from the Hubble Space Telescope Key Project to measure the Hubble constant". The Astrophysical Journal. 553 (1): 47–72.
[9] Riess, A. G. et al., (1998) "Observational evidence from supernovae for an accelerating universe and a cosmological constant". Astronomical Journal. 116 (3): 1009–38.
[10] Perlmutter, S., et al. (1999). "Measurements of Omega and Lambda from 42 high redshift supernovae". Astrophysical Journal. 517 (2): 565–86.
[11] https://lambda.gsfc.nasa.gov/product/map/current/map_bibliography.cfm
[12] Freedman, W. L., (2017) “Cosmology at a crossroads”. Nature Astronomy, 1, 0169
[13] Planck Collaboration, Ade, P. A. R., et al. (2014) “Planck 2013 results. XVI. Cosmological parameters”, Astronomy & Astrophysics, 571, A16
[14] Riess, A. G., et al. (2011) “A 3% Solution: Determination of the Hubble Constant with the Hubble Space Telescope and Wide Field Camera 3”. The Astrophysical Journal, 730, 119
[15] Riess, A. G., et al. (2021), arXiv:2112.04510
[16] Riess, A. G. et al., (2019), “Large Magellanic Cloud Cepheid Standards Provide a 1% Foundation for the Determination of the Hubble Constant and Stronger Evidence for Physics beyond ΛCDM” , The Astrophysical Journal, 876, 85
About the Author
Yun Chen, Associate Research Fellow, National Astronomical Observatory, Chinese Academy of Sciences, Ph.D. in Department of Astronomy, Beijing Normal University, ph.D. in 2012, visiting Kansas State University for one year through the State School of The People's Republic of China, postdoctoral research at Tsinghua University in Hsinchu, Taiwan and National Astronomical Observatory of the Chinese Academy of Sciences, currently researching cosmology applications of lyman-alpha line clusters, strong gravitational lens cosmology, dark energy theory and observational testing.
Yanchuan Cai, a researcher at the University of Edinburgh and the Royal Society University, received his Ph.D. from Durham University in 2009, and has been engaged in postdoctoral research at the University of Pennsylvania, Durham University in the United Kingdom, and the University of Edinburgh, mainly involving statistical methods and observations of the large-scale structure of the universe.
Rotating Editor-in-Chief: Ran Li
Editor-in-charge: Yuan Fengfang
Editors: Zhao Yuhao, Qiqi
National Astronomical Journal of China, December 2021
#预售 (scheduled to ship on January 4) #
Take stock of the 10 major advances in astronomy in 2021