Synopsis
On October 4, 2021, the high-profile Nobel Prize in Physiology or Medicine was finally unveiled, awarded to David Julius and Ardem Patapoutian in recognition of their discovery of the Transient receptor potential ion channel, revealing the mysteries of our perception of the world.
Back in 2012, Robert J. Lefkowitz and Brian K. Kobilka won the Nobel Prize in Chemistry for "G protein-coupled receptor research." In 2003, Peter Agre and Rodrick Mackinnon were awarded the Nobel Prize in Chemistry for their work on ion channels. These three Nobel Prizes, coincidentally they are all separated by 9 years, are all favored by membrane protein research.
As the main physiological sensor, membrane proteins cover many receptors such as vision, smell, temperature and pressure perception, and are involved in the wide range of physiological processes and the high degree of attention received by the scientific community, making it an important target in the field of drug development.
More than 50% of all drugs currently on the market are composed of human membrane proteins. GPCRs (G-protein coupled receptors) are the largest family of membrane proteins in the human body.
Next, Xiaobian will focus on the structure of GPCRs, signaling pathways, and the development pattern of targeted drugs at various stages. ACROBiosystems breaks through the difficulty of preparing GPCRs antigens, and can provide full-length GPCRs antigens to help antibody development, which is recommended.
<h1 class="pgc-h-arrow-right" data-track="7" > GPCRs structure</h1>
The common molecular structure of GPCRs consists of 7 transmembrane α helices that divide the receptor into extracellular N-terminus, intracellular C-terminus, 3 extracellular rings, and 3 intracellular rings. The extracellular ring contains two highly conserved cysteine residues that can stabilize the spatial structure of the receptor by forming disulfide bonds. There are G protein binding sites on the intracellular ring. Taking CCR5 as an example, the classical GPCRs topology is shown in the following figure.
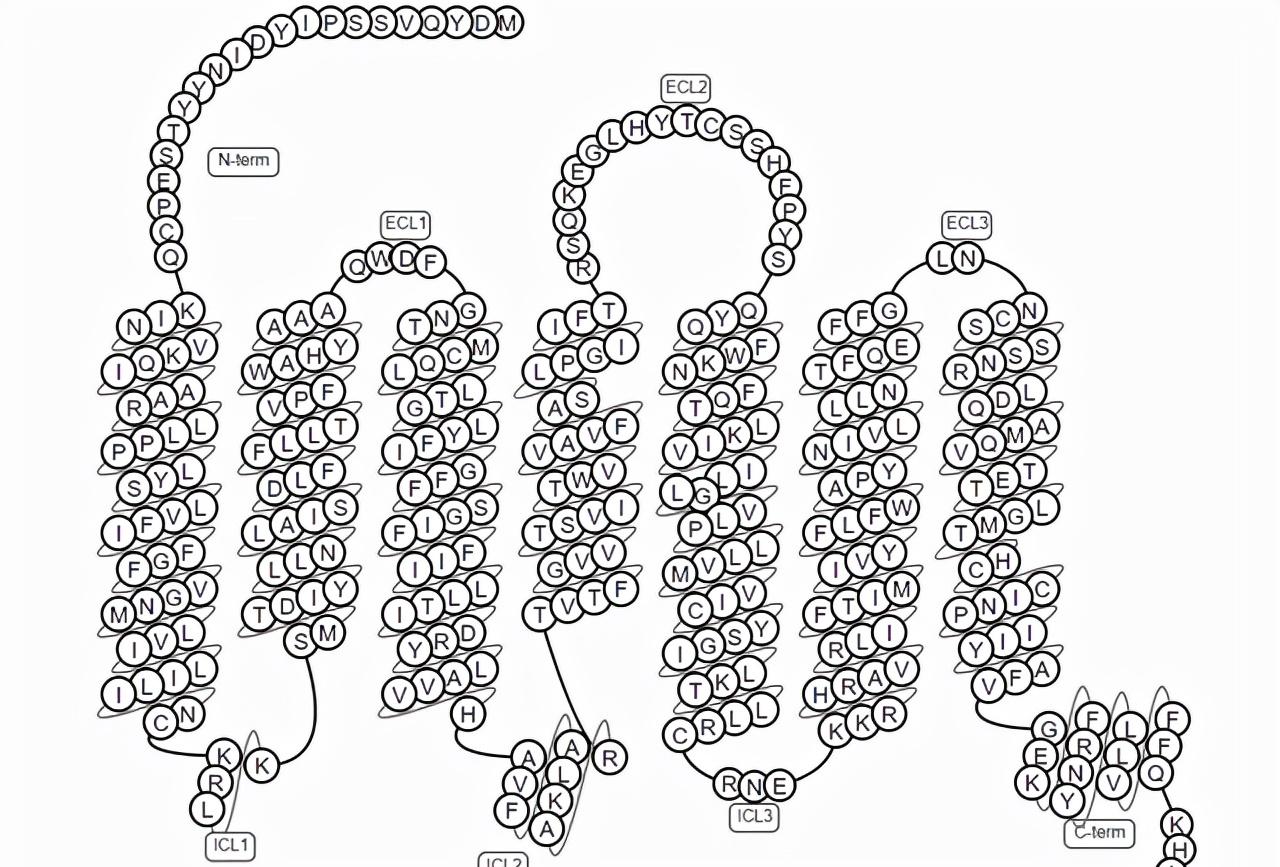
Figure 1. Schematic diagram of the classic GPCRs topology
<h1 class="pgc-h-arrow-right" data-track="11" > signaling mechanism for GPCRs</h1>
G protein is a class of proteins that are coupled with GPCRs and can bind to guanylate during signal transduction, located in the cytoplasm of cell membranes, and are trimers composed of three subunits of α, β and γ. The main function of G protein is to activate the effector protein through changes in its own conformation, thereby realizing the transmission of signal from extracellular to intracellular. In the resting state, GPCRs do not bind to ligands, and αβγ trimers in the cytoplasm bind to GDP (Guanosine diphosphate) and are isolated from the receptors, inactive. When ligands bind to GPCRs, the sites where GPCRs bind to G proteins α subunits are exposed and interact with them, resulting in alterations in the conformation of α subunits, weakened affinity with GDP, and enhanced affinity with GTP (Guanosine triphosphate). At this time, the GDP-αβγ complex, with the participation of Mg, exchanges the combined GDP with gtp in the cytoplasm to form a GTP-αβγ complex. The G protein is then activated and separated from the receptor, simultaneously disintegrating into two parts, GTP-α and βγ, which diffuse freely along the cell membrane and directly interact with the effector protein downstream of the cell membrane, leading to effector activation and initiation of a second messenger cascade, completing the signal transmission from the extracellular to the intracellular. When the ligand and the receptor are unbound, the α subunit itself has GTP enzyme activity, which can promote the hydrolysis of GTP to GDP, and then form a G protein trimer with the βγ subunit to restore the original resting state. G protein downstream signaling proteins are usually ion channels or enzymes that bind to membranes, usually adenylate cyclase and phospholipase C.
Figure 2: GPCRs signal path
GPCRs exist in all cells in the body, can be coupled to a variety of ligands, including ions, neurotransmitters, sugars, lipids, endogenous polypeptides, proteases and other biological macromolecules, by activating G proteins and other signaling molecules to participate in many physiological processes, including muscle contraction and relaxation, nerve signaling, immune system regulation and overall metabolism of the body. GPCRs are also the largest family of medicines in the human body, involving diseases mainly including tumors, autoimmunity, nervous system diseases, cardiovascular diseases, etc., therefore, GPCRs are excellent drug targets for the treatment of a variety of diseases.
<h1 class="pgc-h-arrow-right" data-track="15" > advances in drug development targeting GPCRs</h1>
GPCRs have been in the spotlight as pharmacological targets. In 2011-2015, total drug sales against GPCRs were approximately $890 billion, accounting for 27% of the global therapeutic drug market share. In addition to about half of the olfactory receptors in GPCRs, 227 (57%) of non-olfactory receptors remain untapped and still have broad therapeutic potential, especially in genetic and immune system diseases.
In 2017, survey data showed that there were 481 therapeutic drugs targeting GPCRs, accounting for about 34% of all FDA-approved drugs, involving a total of 107 unique GPCRs. About 320 of these drugs are in clinical trials, and about 35 percent target 64 potential new GPCRs targets.
In the field of drug development targeting GPCRs, GPCRs antibodies have unique advantages over small molecule drugs:
The clearance in the body is lower, the duration of action is longer, and the corresponding frequency of administration is lower;
The selectivity of antibodies is significantly better than that of small molecules;
Due to the barrier of the blood-brain barrier, antibody drugs cannot enter the central nervous system, so for GPCRs expressed in the peripheral and central nervous systems, if only the peripheral part of the drug is designed, therapeutic antibodies can be developed, so that the drug is mainly distributed in the peripheral region and reduces the toxic side effects on the central nervous system.
Figure 3: Clinical drug target distribution of GPCRs
At present, there are about 14 GPCRs targeting antibodies in the active state worldwide, and the targets are CCR4, CGRPR, CCR5, GCGR, GPRC5D, GLP1R, C5AR, CB1, S1PR1, CCR8, CCR7, GPR49, and AGTR1. A representative drug is Leronlimab, a humanized IgG4 monoclonal antibody that targets CCR5 and is an inhibitor of HIV virus entry. By masking CCR5, HIV (R5) subtypes are blocked from entering healthy T cells, thereby protecting these cells from viral infection.
Drug Information For Targeting GPCRs (Clarivate Database)
<h1 class="pgc-h-arrow-right" data-track="25" >ACROBiosystems helped target GPCRs antibodies</h1>
The first step in the preparation of antibodies is antigen immunity, and the first step of immunity is the preparation of antigens. Due to the limited outcrops of GPCRs targets, low cell surface expression, protein aggregation and other factors, it is extremely difficult to obtain biologically active soluble GPCRs antigens, which has also become a bottleneck in the development of GPCRs drugs. To obtain full-length natural GPCRs antigens for drug development, two bottlenecks need to be broken:
Increase in expression. Most membrane proteins are expressed very little on the cell membrane, and some are even expressed only for a short period of time in the cell cycle. At the same time, unlike secretory proteins, the expression and display of membrane proteins is limited by the membrane area, and because many membrane proteins are involved in the function of material transport and signal transmission, excessive expression on the cell membrane will cause irreversible damage to the cell, and these properties of membrane proteins greatly limit their expression. Therefore, in order to obtain sufficient amounts of full-length membrane proteins for immunity and drug development, it is necessary to design and optimize in terms of expression range, expression system, culture conditions, etc. Even so, the cost of obtaining milligram-level membrane proteins is still much higher than that of soluble proteins.
Maintain the homogeneity and activity of membrane proteins during expression and purification. The transmembrane domain of membrane proteins is highly hydrophobic, so unprotected exposure to water will lead to non-specific protein aggregation or even denaturation, so the hydrophobic properties of the surrounding environment of membrane proteins need to be maintained at all times during enrichment and purification of membrane proteins. At present, the commonly used method is to extract the membrane protein from the phospholipid bilayer cell membrane by detergent and form a micelle to maintain its natural conformation and functional activity as much as possible, and there are also methods such as building nanodiscs, virus-like particles (VLP), polymer lipid particles (PoLiPa) for membrane protein reconstruction and functional studies.
In order to help target GPCRs drug research, ACROBiosystems has specially built VLP, membrane protein-detergent, Nanodisc platform solutions, providing full-length GPCRs proteins, such as GPR5D, CCR5, CCR8, etc., which can be used in immunity, ELISA, SPR, BLI and other scenarios.
In addition to GPCRs, ACROBiosystems can provide a full range of full-length multiple-membrane proteins, including four-time transmembrane proteins CD20, Claudin 18.2, five-time transmembrane protein CD133, and more full-length proteins are under development, so stay tuned and consulted.
bibliography
1. Dong HF, Wigmore K, Carrington MN, Dean M, Turpin JA, Howard OM. Variants of CCR5, which are permissive for HIV-1 infection, show distinct functional responses to CCL3, CCL4 and CCL5. Genes Immun. 2005 Oct;6(7):609-19. doi: 10.1038/sj.gene.6364247. PMID: 16015368; PMCID: PMC1369982.
2. Hanlon CD, Andrew DJ. Outside-in signaling--a brief review of GPCR signaling with a focus on the Drosophila GPCR family. J Cell Sci. 2015 Oct 1;128(19):3533-42. doi: 10.1242/jcs.175158. Epub 2015 Sep 7. PMID: 26345366; PMCID: PMC4610211.
3. Hauser AS, Attwood MM, Rask-Andersen M, Schith HB, Gloriam DE. Trends in GPCR drug discovery: new agents, targets and indications. Nat Rev Drug Discov. 2017 Dec;16(12):829-842. doi: 10.1038/nrd.2017.178. Epub 2017 Oct 27. PMID: 29075003; PMCID: PMC6882681.
4. Wiseman DN, Otchere A, Patel JH, Uddin R, Pollock NL, Routledge SJ, Rothnie AJ, Slack C, Poyner DR, Bill RM, Goddard AD. Expression and purification of recombinant G protein-coupled receptors: A review. Protein Expr Purif. 2020 Mar;167:105524. doi: 10.1016/j.pep.2019.105524. Epub 2019 Oct 31. PMID: 31678667; PMCID: PMC6983937.
5. McLean MA, Gregory MC, Sligar SG. Nanodiscs: A Controlled Bilayer Surface for the Study of Membrane Proteins. Annu Rev Biophys. 2018 May 20;47:107-124. doi: 10.1146/annurev-biophys-070816-033620. Epub 2018 Mar 1. PMID: 29494254; PMCID: PMC6370528.
6. Ho TT, Nguyen JT, Liu J, Stanczak P, Thompson AA, Yan YG, Chen J, Allerston CK, Dillard CL, Xu H, Shoger NJ, Cameron JS, Massari ME, Aertgeerts K. Method for rapid optimization of recombinant GPCR protein expression and stability using virus-like particles. Protein Expr Purif. 2017 May;133:41-49. doi: 10.1016/j.pep.2017.03.002. Epub 2017 Mar 3. PMID: 28263854.
7. Hothersall JD, Jones AY, Dafforn TR, Perrior T, Chapman KL. Releasing the technical 'shackles' on GPCR drug discovery: opportunities enabled by detergent-free polymer lipid particle (PoLiPa) purification. Drug Discov Today. 2020 Aug 21:S1359-6446(20)30337-8. doi: 10.1016/j.drudis.2020.08.006. Epub ahead of print. PMID: 32835806.
Please pay attention to "ACROBiosystems Official", if you have any questions, please leave a message or private message.