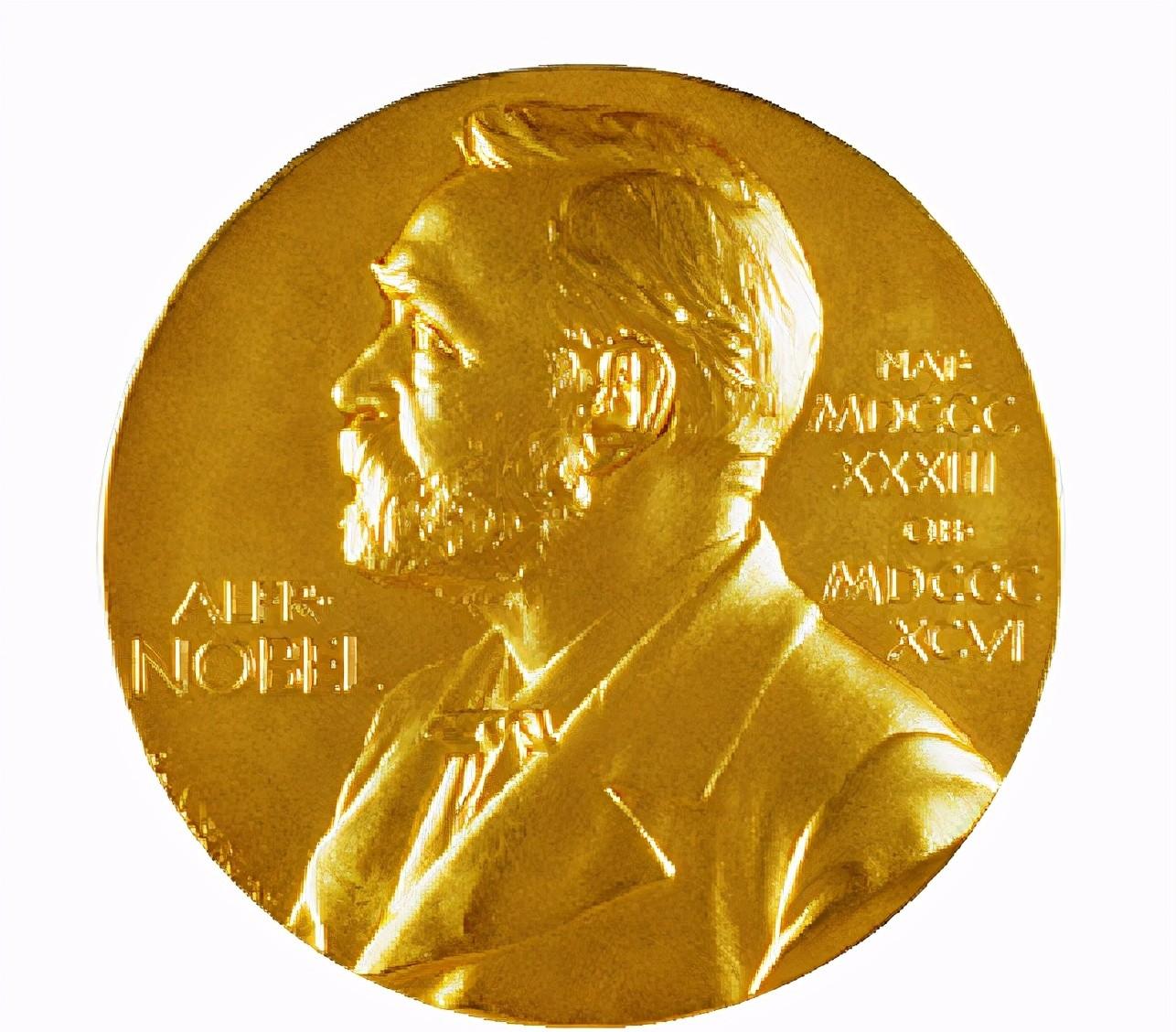
Three scientists won the prize
At 11:45 a.m. Swedish time on October 4, 2017 (17:45 Beijing time), Göran K. Hansson, Secretary-General of the Royal Swedish Academy of Sciences, announced that the 2017 Nobel Prize in Chemistry would be awarded to Jacques Dubochet of the University of Lausanne in Switzerland, Joachim Frank of Columbia University in the United States, and Richard Henderson of the University of Cambridge in the United Kingdom Henderson), in recognition of their contributions to "developing cryo-electron microscopy for the determination of high-resolution structures of biological macromolecules in solution." The three winners will be divided equally in prize money of SEK 9 million (about US$1 million).
Introduction of winners
Jacques Dubochet was born on 8 June 1942 in Aigle, Switzerland. Winner: University of Lausanne, Switzerland.
Nobel Laureate: Jacques Du Pochet
Jacques Du Bochet was born in Egger, Switzerland. He studied physics at the University of Lausanne and later molecular biology at the University of Geneva. In 1973, he completed his doctoral dissertations in biophysics at the Universities of Geneva and Basel. From 1978 to 1987 he worked at the European Molecular Biology Laboratory in Heidelberg and later returned to work at the University of Lausanne.
In the early 1980s, Jacques Du Bochet succeeded in rapidly cooling water to obtain a glassy solid instead of the usual crystalline form of ice. This enables biological samples to be successfully applied to electron microscopy observation.
Joachim Frank was born on 12 September 1940 in Siegen, Germany. Winner's work unit: Columbia University, USA.
Nobel Laureate: Achim Frank
Achim Frank was born in Siegen, North Rhine-Westphalia, Germany. After studying at the University of Freiburg and the University of Munich, he received his Doctorate degree from the Technical University of Munich in 1970. Frank has worked at several institutions in the United States and Europe, including the Wadsworth Center, the New York State Department of Health, the State University of New York at Albany, the Howard Hughes Institute of Medicine, and Columbia University, and has been at Columbia University since 2008. Achim Frank is married and has two children.
Between 1975 and 1986, Joachim Frank developed a method to obtain sharp three-dimensional images by algorithmically analyzing and merging blurry two-dimensional electron microscope images. This allows electron microscopy to look at more biomolecules.
Richard Henderson was born on 19 July 1945 in Edinburgh, Scotland. He received his B.A. in Physics from the University of Edinburgh in 1966 and his PhD from the University of Cambridge in 1969. Awarded for work at MRC Molecular Biology Laboratory, Cambridge, UK.
Nobel Laureate: Richard Henderson
Richard Henderson was born in Edinburgh, Scotland. After studying at the University of Edinburgh, he received his PhD from the MRC Molecular Biology Laboratory at the University of Cambridge in 1969. After a stint at Yale University in the United States, he returned to the MRC Molecular Biology Laboratory in 1973 and has been working there ever since.
Richard Henderson successfully advanced the resolution of biomolecules such as bacterial rhodopsin to atomic resolution using the two-dimensional crystal method.
Introduction to research work
< > from optical microscopy to electron microscopy
People are full of curiosity about everything in the world, and it is often said that seeing is believing, and image information is the key to our understanding of everything. But in ancient times, people's observation of things could only be achieved through the naked eye, and the human eye's ability to distinguish was only about 0.1 mm, so the cognition of many things was not comprehensive. About a thousand years ago, people stumbled upon the grinding of transparent crystals or gemstones into "lenses", and found that these lenses can magnify images, which for the first time improved the human eye's ability to observe.
Hooke used a microscope and his drawing of wooden plug cells
By 1590, the Dutchman Jensen had invented the world's first microscope. In 1665, the English scientist R. Hooke Hooke) looked at the plant's peg tissue with a microscope and found that it was made up of many regular small squares, and he drew the observed images and called these small squares cell, which is the cell in English. Later the Dutchman A. Leeuwenhoek Van Leeuwenhock himself learned to grind lenses, build a microscope, and observed the structure of tiny plants and animals, contributing greatly to the development of biology. Since then, biology has developed significantly, and with the help of microscopes, smaller structures can be observed.
Optical microscope
But this kind of microscope, first with light irradiated on the object being observed, and then through the treatment of reflected light, the final enlarged image is obtained, so we generally call it an optical microscope. However, the magnification ability of optical microscopes is limited, and the current optical microscopes can only enlarge objects up to 2,000 times. The resolution capacity of the microscope is about half the wavelength of visible light, and the wavelength range of visible light is 300 to 700 nanometers, so the theoretical minimum resolution of the optical microscope is 0.172 microns, and it is powerless to face the smallest structure.
The principle of microscope magnification
With the development of science and technology, optical microscopes can no longer meet the needs of scientific research. After the 20th century, quantum mechanics has made significant progress, and people's understanding of microscopic particles has come one step closer. Electrons have the characteristics of wave-particle duality, with wave phenomena such as interference and diffraction. By accelerating the electrons, when the acceleration voltage is 50 to 100 kV, the wavelength of the electrons at this time is 0.0053 to 0.0037 nanometers, which is much smaller than the wavelength of visible light. If electrons can be used as the medium of the microscope, the resolution can be greatly improved, and people can observe finer structures.
In 1931, the Germans Knorr-Bremse and Ruska modified a high-voltage oscilloscope using a cold cathode discharge electron source and three electron lenses, obtaining images magnified by dozens of times, proving the possibility of electron microscopy magnification imaging. After Ruska's modification, the resolution of the electron microscope increased to 50 nanometers, which was about ten times the resolution capacity of the optical microscope at that time, and the electron microscope began to receive attention. Electron microscopy allows people to see viruses and some macromolecules, and the scanning tunneling microscope invented later allows people to see the structure of atoms.
The composition of the electron microscope consists of three parts: a mirror barrel, a vacuum system and a power cabinet. The lens barrel includes components such as electron sources, electronic lenses, sample holders, phosphor screens, and detectors. Among them, the electronic lens is the most important component. The convex lens in a light microscope allows the beam to gather, and the electron lens causes the electron beam to gather. Modern electron microscopes mostly use electromagnetic lenses, which cause electrons to accumulate through a strong magnetic field.
Comparison of optical microscopy and electron microscopy principles
Projection electron microscopy has basically the same imaging principle as optical microscopy, except that it uses an electron beam as the light source and the electromagnetic field as the lens. Because the penetration of the electron beam is very weak, the specimens used for electron microscopy must be made into ultra-thin sections with a thickness of about 50 nanometers. Transmission electron microscopy is the projection of an accelerated and aggregated beam of electrons onto a very thin sample, and the electrons collide with the atoms in the sample to change direction, resulting in solid-dimensional angle scattering. The size of the scattering angle is related to the density and thickness of the sample, so it is possible to form different light and dark images, which are magnified and focused on imaging devices (such as fluorescent screens, films, and photoreceptor coupling components).
Modern electron microscopy
< ii> Observation of biological materials
Electron microscopy requires bombarding the surface of a substance with high-speed electrons, so it is often suitable for "inanimate" samples. For biomaterials, it is relatively fragile and cannot withstand the indiscriminate bombardment of electrons, so the newly invented electron microscope cannot be used for biological research.
In 1934, Ladislaus Laszlo Marton used electron microscopy to examine biological specimens and wrote an article proposing two possible solutions: using negative staining or using cryogenic cooling of biological materials.
The negative staining method was gradually perfected after the advent of the 1940s, which formed a layer of amorphous film of heavy metal salt ions, and then embedded biomolecules in the membrane. Heavy metal salt ions and biomolecules have different scattering capabilities for electrons, which is reflected in the picture is that white biomolecule samples are scattered in a black background. But the resolution of this method is limited by the particle size of the heavy metal salts. Despite this, at that time, the negative staining method could see structures that could not be seen under ordinary light microscopes, so it still played an important role in the three-dimensional structure of some biomolecules and three-dimensional reconstruction techniques. Negative staining is still widely used in pre-sample screening and initial model construction.
But negative staining can only observe organelle-sized structures, and is powerless for smaller single molecules or molecular complexes.
Marton's second idea was to keep biological samples at low temperatures, so that biological samples could withstand more electron bombardment, and the problem of biological samples dehydrating and deforming in vacuum was solved. But water forms crystalline ice at low temperatures, and crystal ice causes electrons to diffract strongly, causing the detector to collect almost all the signals of crystalline ice, thus masking the signals of biomolecules. At the same time, crystalline ice also affects the natural structure of proteins. Most biomolecules can only maintain their natural spatial structure in aqueous solutions. Therefore, in order for biological samples to remain natural at low temperatures, the problem of crystalline ice must be solved.
Theoretically, the problem of crystalline ice formation can be overcome by rapidly cooling liquid water into a glassy (amorphous state). But theoretical predictions before the 1980s suggest that the cooling rate required for a large number of aqueous phase transitions to form glassy ice is difficult to achieve. Meyer's 1980 study confirmed that droplets of fast-frozen size at the micron level can convert them into glassy ice.
In the 1980s, Jacques Duboche devised a feasible solution for freezing thin water films into glassy thin ice films. The solution containing the sample is dropped in the treated copper mesh, formed by processing a thin water film of nanometers to microns, and then rapidly submerged to -180 ° C temperature (liquid ethane or propane), at which time the thin water film on the copper mesh is quickly frozen into a thin ice film, and the biological sample is also fixed in the thin ice film. Duboche used this method to prepare frozen samples of many different kinds of biomolecules, such as bacteriophages, DNA, viruses, ribosomes, etc. It shows that this method is feasible and has high repeatability.
Preparation method of Jacques Duboche frozen biological samples
< three > increase the resolution of biological samples
Du Bochet's invention of the sample preparation method made electron microscopy biology enter the era of cryo-EM, laying the foundation for the analysis of high-resolution structures. In 1990, Richard Henderson et al. successfully advanced the resolution of bacterial rhodopsin to atomic resolution using the two-dimensional crystal method on the basis of the frozen sample preparation method.
Henderson first used X-ray technology to resolve the structure of biomolecules, but this method required the crystal state of biomolecules, and the crystallization of biomolecules such as membrane proteins was extremely difficult. After many failures, Henderson turned to electron microscopy because electron microscopy does not require biomolecular crystallization to resolve its structure.
The earliest observed rhodopsin
Bacterial rhodopsin is a special protein, which under certain conditions will form a neat layer of purple film, and the purple film is a two-dimensional crystal, because the protein is only neatly arranged on a plane, not stacked in the third direction of the stereoscopic. Henderson et al. took a different approach and directly made a sample of the purple film. That was not yet the concept of frozen samples, Henderson made samples at room temperature, and to prevent the samples from drying out in a vacuum, he added a 0.5% glucose solution to them.
Usually biological samples can only be tested using lower electron doses, resulting in lower image quality. Two-dimensional crystals diffract electrons, which can improve the image quality through software processing. By rotating the sample, the data of the sample at different angles can be obtained, and the three-dimensional structure of rhodopsin can be constructed using the method of crystal analysis, and the technology of chemical crystal analysis is very mature.
By using the above method, Henderson resolved the resolution of this protein membrane structure to 0.7 nm, and saw the transmembrane spiral structure of the membrane protein for the first time. Later, after the development of cryo-electron microscopy technology, Henderson also carried out a large number of studies, and in 1990 further increased the resolution of rhodopsin to 0.35 nm.
Rhodopsin observed in 1990
Henderson's work was not only the first to resolve membrane proteins to atomic-level resolution, but also gave cryo-EM confidence in resolving atomic-level resolution structures. Subsequently, the high-resolution structure of membrane proteins such as photocaping complexes, αβ dimer tubulin, and aquaporin proteins was also resolved.
< four > enables the observation of more biomolecules
However, not all biomolecules can form two-dimensional crystals, and more biomolecules can only exist in solution in the form of individual molecules. Electron microscopy analysis of the three-dimensional structure of a single molecule can only be carried out by the three-dimensional reconstruction method of single-particle cryo-EM.
In the 1970s, Joachim Frank et al. initially studied enhancing the image quality of images at low electron doses. By improving the image quality of the picture (enhancing the signal-to-noise ratio) by means of a homogeneous superposition method, Frank obtained a two-dimensional projected average of glutamine synthase. But the two-dimensional projection of biomolecules at different angles is different, even if the projection in the same direction, the two-dimensional projection produced by different conformations is also different. Projections from different angles of biomolecules need to be categorized separately. Classification can be made by looking for projected feature points or feature regions by comparing them. But looking for sign spots or feature areas in low-quality images is also a challenge. Frank and van Heel solved this problem using a method called multidimensional data analysis.
Electron microscopy diagrams of allomolecules in 2011 (top) and 2016 (bottom).
Reconstructing a 3D structure from a 2D projection requires determining the relative position of each 2D projection in the 3D structure. In 1986, Frank and Radermacher et al. created a method called random cone rotation that could determine the relative position of a two-dimensional projection in a three-dimensional structure.
Frank made a great contribution to the development of electron microscopy biology in theory, and he also developed the electron microscopy processing software SPIDER.
The direct electron detection technology (DED) invented in the early 21st century, compared with the previous CCD, DED does not need to convert the electronic signal into an optical signal, but directly detects the electronic signal, reducing the excess noise introduced during conversion. In 2012, DED was introduced to cryo-EM, using the method of single-particle cryo-EM, and the structure of the transmembrane protein TRPV1 was resolved to 0.38 nm. Today's single-particle frozen electrons, 334kDa protein structure resolution can reach 0.18nm, 64kDa protein structure resolution can reach 0.32nm.
Progress in the development of electron microscopy
To sum up, the process of understanding living organisms is as follows: living organisms - tissues and organs - cells - organelles - biomolecules. Through the naked eye can observe most of the organisms in nature, through the dissection of people understand the tissues and organs of the body, optical microscopy allows people to observe the structure of cells, and electron microscopy allows people to further observe the structure of organelles, until the development of cryo-EM, people can now observe the structure of many biomolecules.
Summarizing the contributions of the three people, Du Bochet's invention of the sample preparation method made electron microscopy biology enter the era of cryo-EM, laying the foundation for the analysis of high-resolution structures. Henderson's work was not only the first to resolve membrane proteins to atomic-level resolution, but also gave cryo-EM confidence in resolving atomic-level resolution structures. Frank made a great contribution to the development of electron microscopy biology in theory, and he also developed the electron microscopy processing software SPIDER.
Resources
[1] https://www.nobelprize.org
Li Zhifei,Gao Ning. Application of Cryo-EM Technology to High Resolution Structure Analysis of Biomolecules——A Brief Discussion of the 2017 Nobel Prize in Chemistry[J].University Chemistry,2018,33(01):1-6.