Silicon-based quantum computers are about to become possible.
Recently, Nature published three papers on the cover, and researchers from three different teams verified the fidelity of the silicon double qubit gate, all of which reached more than 99%, exceeding the fault tolerance threshold of quantum computing. The results confirm that powerful and reliable quantum computing in silicon materials is becoming a reality, helping to build silicon-based quantum devices that are compatible with current semiconductor technologies.
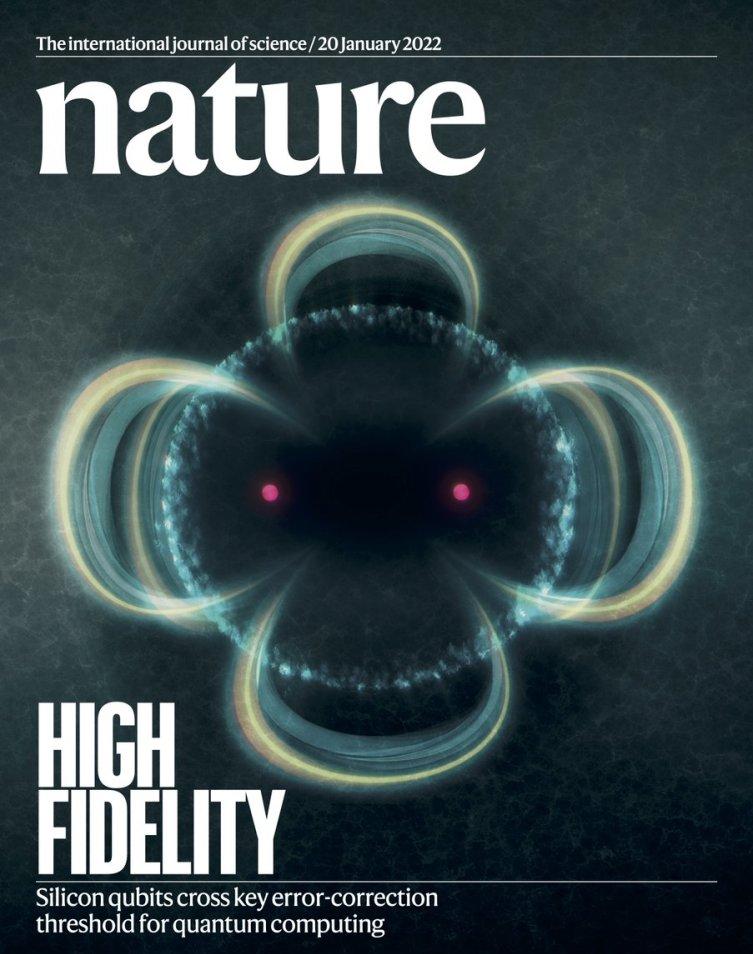
Nature cover, image from Nature
Silicon-based quantum computing using nuclear spin
Nuclear spins are one of the first physical platforms considered for quantum information processing because of their excellent quantum coherence and atomic-scale trajectories. But the potential of nuclear spin in quantum computing has yet to be tapped into a way to connect nuclear qubits to scalable quantum devices and maintain sufficient fidelity to maintain fault-tolerant quantum computing.
A research team at the University of New South Wales in Australia created a double qubit universal quantum logic operation between two nuclear spins formed by a phosphorus donor, which was introduced into a silicon nanoelectronic device through an industry-standard ion implantation method. Using a method called Quantum Gate Set Tomography (GST), they validated the performance of their quantum processor, achieving up to 99.95% single qubit gate fidelity, 99.37% dual qubit gate fidelity, and 98.95% double qubit preparation/measurement fidelity. Three pieces of data suggest that the nuclear spin in silicon is close to the performance required by fault-tolerant quantum computers.
The researchers demonstrated the achievability of general-purpose quantum operations, which means that all the fundamental operations that make up quantum operations, including single-qubit operations and double-qubit operations, can be performed at fidelity above the fault tolerance threshold.
In addition, according to the results of the study, the electron spin itself is a qubit, which can be entangled with two atomic nuclei to form a three-qubit quantum entanglement state with a fidelity of 92.5%. This provides a viable method for achieving a silicon-based quantum computer that uses electron spin and nuclear spin.
Three qubits of quantum entanglement, red dots for the qubits of phosphorus atoms, shiny ellipses for electron spins, image from the University of New South Wales
Silicon-based quantum computing based on electron spin
Achieving more than 99% double qubit gate fidelity has been a major goal for semiconductor spin qubits.
The high-fidelity control of qubits is critical to the reliable execution and fault tolerance of quantum computing. Fault tolerance is the ability to correct errors faster than they occur.
The delft University of Technology research team in the Netherlands used materials formed from stacks of silicon and silicon germanium alloys to create a dual qubit system. Among them, quantum information is encoded in electron spins confined to quantum dots, and ultimately achieves 99.87% single qubit fidelity and 99.65% dual qubit fidelity. Even after adding crosstalk and idling errors from adjacent qubits, the fidelity of the average single qubit gate is still higher than 99%.
The team used a variational quantum-based solving algorithm (VQE) to perform the operation and achieve more than 99% double qubit gate fidelity. This facilitates the role of semiconductor qubits in fault-tolerant quantum computing, which may be applied to medium-sized quantum devices in the future.
Dual qubit devices and symmetric arithmetic points
Quantum computing of silicon spin qubits
The research team at the Rikyochemical Research Institute of Japan used the same material stack produced by delft's team, in a silicon spin qubit, through a gradient field induced by micromagnets and fast electrical control coupled with tunable double qubits, and used the double qubit Deutsch-Jozsa algorithm and the Grover search algorithm to achieve 99.8% single qubit fidelity and 99.5% double qubit fidelity.
Fault-tolerant quantum computers rely on quantum error correction, which requires universal qubit gate fidelity to exceed the fault tolerance threshold (99%). Historically, among the many qubit platforms, only nitrogen-vacancy centers in superconducting circuits, ion traps, and diamonds could meet this requirement.
The electron spin qubits in silicon are promising for the development of large-scale quantum computers due to their nanofabrication capabilities. However, due to its slow operation, its dual qubit gate fidelity has been limited to 98%.
The results of these studies allow the silicon spin qubit to compete with superconducting circuits and ion traps in terms of general-purpose quantum control performance. This means that silicon quantum computers are capable of performing high-precision quantum computing.
Dual qubit system
The study also shows that silicon quantum computers, like superconducting circuits and ion traps, are one of the best candidates for large-scale quantum computers.
Previously, the mainland research team has also discovered the advantages of silicon-based spin qubits. Academician Guo Guangcan's team at the University of Science and Technology of China collaborated with Researchers from the United States and Australia and Origin Quantum to achieve ultra-fast manipulation of silicon-based spin qubits with a spin-flip rate of more than 540MHz – the highest value reported internationally, and the research paper was published in the journal Nature Communications on January 11.