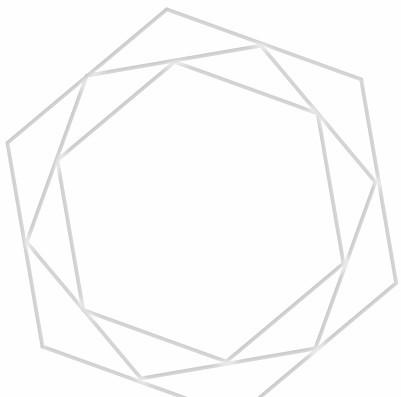
Follow the voice of the storm
Elevate your thinking
Guide
Einstein proved precisely that truly great science is synthetic and intersecting.
READING
In 1905 Einstein published several important papers, so that year was known in physics as Einstein's "year of miracles" (annus mirabilis). 100 years later, in 2005, Philip Ball, a former consultant editor of the British journal Nature and a well-known popular science writer, wrote an article entitled "Claiming Einstein for Chemistry", which was published in the Chemistry World magazine of the Royal Society of Chemistry. In 2005, physical chemist Wang Hongfei was invited by the Chemistry Department of the National Natural Science Foundation of China to translate this article into Chinese.
Einstein has always been thought of as a genius in physics, and few seem to call him a chemist. Many of the ideas he introduced were unexpected for many "orthodox" physicists of the time. Through Ball's article, we can understand that it may be the physical chemistry of Einstein's thought that sets him apart from the average physicist.
Of course, Einstein's shortcomings as a physicist may also come from his background in physical chemistry. Physicists generally consider themselves superior to chemists and biologists, and Einstein proved that truly great science is comprehensive and intersecting, not limited to one discipline.
Author | Philip Bauer (Former Consultant Editor, Nature)
Translated by | Wang Hongfei (Professor, Westlake University)
Was Albert Einstein essentially a chemist? Today, he is always used as the standard prototype for theoretical physicists, who often write on blackboards mysterious and illegible formulas about the nature of space and time. But Einstein's early work, largely concerned with the molecular nature of matter, was firmly rooted in concrete, reality-perceptible things. Not only physicists, but also chemists should commemorate this "Einstein Year" that remembers his most important discovery.
In fact, when Einstein published his special theory of relativity in 1905, what surprised his contemporaries was perhaps not the revolutionary conclusion of the theory, but the fact that this amazing work in modern physics came from a promising young man who was entering the field of Physical Chemistry.
01
Einstein's doctoral dissertation in physical chemistry
In his doctoral dissertation submitted to the University of Zurich in Switzerland in July 1905, Einstein proposed a new method for calculating the Avogadro constant and the size of molecules. That same month, he published a paper showing that the chaotic and irregular motion of tiny particles in liquids observed under the microscope— the so-called Brownian motion — is caused by collisions of solvent molecules.
In the articles published in this "year of miracles," Einstein himself believed that there was only one that was truly revolutionary. In this article, he attempts to explain how metals interact with light by introducing the concept of quanta, that is, discontinuous energy packs. All in all, this is a job that any physical chemist would be proud of.
Now, starting from Einstein's theory of relativity, people have obtained important and incredible concepts such as black holes and time travel through space-time holes; and from quantum theory, we have also obtained the concepts of parallel universes and bubble-like space-time that are infinitely bifurcated, and it is difficult to understand how ordinary these theories come from and how ordinary they seem on the surface.
The theory of relativity was proposed to solve the difficulties encountered in applying James Clark Maxwell's theory of electromagnetism to moving objects, and Einstein's 1905 article began with only magnets and coils.
But the main reason for proposing Einstein to be a chemist (even a little insincerely) has a deeper reason than the above. At a time when Einstein made his original contribution, there was no strict demarcation between physics and chemistry. At the time, these boundaries were only mentioned as physicists and chemists struggled to make demands on them for new areas of research such as radioactivity and nuclear science.
At the time, scientists were still debating the existence of atoms and molecules proposed by John Dalton, or simply a concept invented for the convenience of teaching and explanation, and the central principle used by chemists like the periodic table of elements was still waiting for the explanation of the quantum theory that would emerge later.
02
Interdisciplinary studies
Einstein himself did not care about the boundaries between these disciplines. This is just like Faraday and James Maxwell, who preceded him 100 years ago. And Einstein was the one who connected these two people in time and intellect.
Einstein's later pursuit of a unified theory of fundamental forces was nothing more than a simple continuation of his earlier work on liquid and intermolecular forces through Newton's theoretical model of matter dynamics.
Newton speculated that the forces acting on the microscopic scale between atoms and the forces that interact on the cosmic scale between stars and planets have the same essence. In two early papers published in 1901 and 1902, Einstein inherited This Idea of Newton and explored the common origin of gravity and intermolecular forces. In 1901, at the age of 21, Einstein wrote: "It is a great feeling to be able to recognize the inner unity between complex phenomena of things that are completely different in our immediate senses." ”
It is no surprise, therefore, that when Einstein was fascinated by Maxwell's theory of electromagnetism at a very early age, his first academic achievement also continued Maxwell's other major contribution to the science of matter— the theory of the motion of gases.
In 1879, Johann Didric van der Valls of the Netherlands extended Maxwell's theory to the treatment of liquids, and Einstein's 1901 paper, published in Annalen der Physik, pursued the same theme that had sparked van der Wals's interest, namely the role of intermolecular forces in capillaries.
Einstein hoped to expand this work to a doctoral dissertation submitted to the University of Zurich, but to no avail, so he accepted a job at the Swiss Patent Office in Bern in April 1901. It is very telling that Einstein's father, out of concern for his son's future, chose to turn to the famous physical chemist William Ostwald for help.
This is the clue to Einstein's 1905 doctoral dissertation on molecular size. Prior to this, there were several methods of determining the size of molecules, the most reliable of which was based on the theory of gas motion.
For example, in 1865 Gypsy chemist Joseph Locksmidt proposed a method of comparing the densities of different liquids and gases, concluding that the diameter of the "air molecule" was about 1 nanometer. From this, Locksmidt could calculate the Avogadro constant, the number of molecules of 1 molar matter. This constant has therefore been referred to as locksmidt's constant in German-speaking countries.
The difference is that Einstein invented a method that can accurately calculate the size of molecules based solely on the properties of liquids. Van der Vals has determined that molecular size is important for understanding the properties of liquids, and it is precisely because of the size of the molecules that he applied the theory of gas motion to the treatment of liquid matter.
03
Movement of particles
Einstein's doctoral thesis studied the motion of particles. This is closely related to his interest in molecular diffusion research. The German chemist Walter Nernst pioneered the study of molecular diffusion, and he also published a paper on Brownian motion in the same year. Stokes' law relates the velocity of particles in a fluid to the viscosity of the fluid. Based on Stokes' law, Einstein derived the equation for the diffusion coefficient of solute molecules in solvents. Einstein's equation contains both the Avogadro constant and the radius of the solute particle, and he solved the equation by calculating the change in the viscosity of solute dissolved in the fluid through the theory of fluid mechanics.
Using experimental data from an aqueous solution of sugar, Einstein calculated that the radius of the sugar molecule was 1 nanometer and came up with the Avogadro constant of 2.1x1023 molecules per mole. It was not until 1909 that the French physicist Jean Perrin more accurately measured the Avogadro constant, indicating that Einstein's value was too small, that Einstein was forced to recheck his calculations.
Einstein couldn't detect anything wrong, so he asked his student Ludwig Hopff to scrutinize it. This made Hopf one of the very few who had the privilege of being able to find errors in Einstein's mathematics. After Hopf corrected an erroneous equation, Einstein's method yielded 6.56x1023, which not only corresponds to Perrin's numbers, but is also very close to 6.02x1023 today.
04
Prove the existence of atoms
Einstein's interest in measuring molecular sizes had deeper scientific goals. He realized that some prominent scientists, including William Ostwald and Ernst Maher, were skeptical of the true existence of atoms and molecules. It is easy to think today that these anti-atomists are unreasonable, but at the turn of the 19th and 20th centuries, there was not a single direct evidence of the correctness of the atomic theory of matter. Most physicists and chemists take atomic theory for granted. But Maher points out that only bad science assumes the existence of entities that cannot be perceived.
Einstein was convinced of the existence of atoms, but he wanted some form of confirmation. He said we need evidence that it "guarantees the existence of atoms of a certain size."
He recognized that this evidence might be obtained from the phenomenon of Brownian motion; or, from the random motion of microscopic particles suspended in liquids. This random motion is hinted at to be related to the motion of particles observed by botanist Robert Brown in 1828.
When Brown observed pollen particles bouncing around irregularly in the water, he initially thought it was a manifestation of the "vitality of life" that existed in an organism, which many people thought existed at the time. But he soon discovered that "dead" pollen particles behaved in the same way, and his observations gave rise to a variety of theories in the 19th century, even involving convection and electricity. But none of these theories are satisfactory.
05
Random molecular motion
Einstein's approach to this problem relies on the notion that heat, established through the theory of gas motion, is the result of the motion of random molecules. It had been hypothesized that although the random motion of molecules had a high rate, since suspended dust or pollen particles were much more massive than individual molecules, the collision effect of molecules on these large particles was negligible, just like a meteorite hitting the Earth.
But Einstein showed that the statistical imbalance in the number of molecules hitting a micron-sized particle from different directions does make the particle move, and the cluttered motion due to the thermal motion of the molecule can indeed be significant enough to be observable under the microscope.
The randomness of this motion causes the particle to make a diffusion motion in the liquid: if it follows its motion over a period of time, it will reach a different place than it did at the beginning. Einstein could calculate this function of mean displacement over time and predict that a 1 mm-sized particle can move about 6 millimeters in 1 minute in water.
This quantitative prediction is crucial: it provides a way to verify the correctness of Einstein's theory. If the theory is quantitatively confirmed, it will be difficult to deny the correctness of the molecular image of matter. This image is the basis of the entire theory of motion. In other words, molecules must be real. Einstein concluded his 1905 article with the hope that "[experimental] researchers will soon succeed in solving the problems raised here."
Many people have tried experiments, but this experiment is very difficult, mainly due to the difficulty of ensuring that the liquid has a constant and uniform temperature in the experiment. Until 1908, no one had been able to obtain quantitative evidence of Einstein's theory, and he himself began to despair, believing that it was impossible to accurately study Brownian motion. To his delight, Perrin accepted the challenge and confirmed the correctness of the theoretical predictions this year. For this work, Perrin was awarded the 1926 Nobel Prize in Physics.
It's no secret that Einstein spent his life uneasy about certain fundamental properties of quantum theory, especially the way in which quantum theory seems to confer opportunity and uncertainty on matter's behavior.
To some extent, he resembled Planck, using the quantum description of matter as a convenient tool for understanding certain specific properties of matter, such as the photoelectric effect and the heat capacity of solids, and on the other hand speculating that there might be a more fundamental theory of certainty beneath it.
06
The core problem of quantum chemistry
The quantum nature of light and energy has central value for chemistry. It illustrates how matter interacts with light, for example, why grass is green and why the sky is blue. It also provides the basis for all spectroscopic methods, allowing us to decipher the structure of molecules.
Nils Bohr, Arnold Sommerfeld, and Wolfgang Pauli showed how quantum models of atoms explain the structure of the periodic table and the properties of elements; while Fritz London, Linus Pauling, and others developed quantum images of chemical bonds between atoms to explain the shape and properties of molecules.
Today, it is impossible to imagine that chemistry can leave quantum theory. Quantum theory has been used to explain and predict everything from the catalytic behavior of metals to stereoscopic behavior of organic synthesis. This aspect of Einstein's work has had an impact on chemistry beyond all other fields of science.
Critics might argue that even if Einstein had started his work in the name of chemistry, we hardly remember him today. The chemist's quantum theory may have been triggered by his work on the photoelectric effect, but shouldn't quantum theory owe more to the painstaking development of Bohr, Schrödinger, and Heisenberg after him, rather than just Einstein's quantization of light? And, didn't his greatest theory of relativity become the language of an astrophysicist, not a chemist? However, this is not so certain.
Relativity is also very important in chemistry. Special relativity states that when an object moves at nearly the speed of light, its mass increases (and at the same time, from the perspective of a relatively stationary observer, it also becomes shorter and lives longer). In heavy atoms, the electrostatic mutual attraction between electrons in the inner orbit and highly charged nuclei causes the electrons to travel very fast, resulting in a relativistic effect: the average velocity of electrons in the innermost layer of a uranium atom is about two-thirds of the speed of light.
07
Orbital electrons
These relativistic electrons become heavier in mass, thus bringing their orbits closer to the nucleus. This further increases the pull of the inner electron shielding nucleus on the outer electrons, so the orbitals of the outer electrons expand and the energy decreases. In this way, the relativistic effect readjusts the electron level of the atom.
This phenomenon is not as bizarre and rare as you might think. If it were not for the relativistic effect, gold would have looked like silver; the reddish color of gold was because it absorbed blue light, and this was due to the relativistic displacement of the electron bands of gold atoms.
Compared with the phenomenon of cosmic gravitational lensing or atomic clock slowdown to any extent, this is indisputably a more far-reaching demonstration of Einstein's theory. For thousands of years, gold has had a lofty cultural status and cultural symbolism, because the metal has been associated with the sun since time immemorial.
Similarly, the relativistic effect gives mercury a low melting point, which not only gives it enormous technical importance, but also gives the metal some sort of mysterious connection to water and the moon culturally.
In recent years, relativistic effects in atoms have become even more important as new superheavy elements are synthesized through the collision of particle beams. Synthesizers of the new elements began to study whether extreme relativistic effects on changes to the electronic structure of these atoms had begun to disrupt the sequential property changes in the periodic table. Even at this frontier of modern chemistry, it is impossible to ignore Einstein's legacy.
Notes
08
Blackbody radiation and quanta
Blackbody radiation is electromagnetic radiation from hot objects capable of absorbing all light. Blackbody radiation has a fairly wide wavelength, but the wavelength of the maximum intensity depends on the temperature of the blackbody: the higher the temperature, the shorter the wavelength. The Prussian physicist William Wien revealed this phenomenon in 1893.
Therefore, the metal wire in the bulb or an electric heater is first dark red, then yellow, and finally white or light blue during the process of being gradually heated. Before it emits visible light, you can feel the heat generated by infrared radiation. At the turn of the 19th and 20th centuries, this common phenomenon was not explained by anyone.
Among those trying to explain was Einstein's doctoral supervisor at the University of Zurich from 1901 to 1902, Heinrich Friedrich Weber.
The other man was Max Planck. He began to derive Venn's relationship between temperature and wavelength through the statistical mechanics method developed by Maxwell and LutherWich Boltzmann in the theory of gas dynamics.
Planck used a series of charged oscillators to represent atoms in black bodies and calculate the electromagnetic energy radiated. His initial calculations seemed to conform to Vane's law; but then experimentalists discovered that Van's law no longer holds at high temperatures.
Planck found that his theoretical predictions could match experimental observations by correcting his theory. This correction requires the assumption that each oscillator has a discontinuous energy E proportional to the oscillator frequency. He proposed the relationship between E = hv , h now known as Planck's constant.
For Planck, this hypothesis was nothing more than a mathematical game to make his theory conform to the results of the experiment. But when Einstein began studying Planck's blackbody radiation work in 1904, he explained it more realistically. Light, he said, has a piece-by-piece energy given by Planck's formula. He called these energy blocks quanta. He claimed that light is quantized.
Einstein knew the proposal was controversial and even intolerable. But he argues that his hypothesis could explain the photoelectric effect observed by Philip Leonard in 1902. Leonard discovered that light emitting electrons when it hit metal. If light is quantized, then it knocks electrons out of the metal when the individual quantum energy exceeds the energy needed to move electrons out of the metal, regardless of the intensity of the light.
This blatantly challenges intuition: one would naturally expect that a stronger light would inject more energy into the metal, so that whatever wavelength it was, it would spew electrons out of the metal. According to Einstein's hypothesis, the electron energy ejected will not depend on the intensity of the light, but on the wavelength of the light, which determines the size of the quantum packet. This is exactly what Leonard's experiments have found.
For his explanation of the photoelectric effect, Einstein won the 1921 Nobel Prize in Physics.
09
Relativistic effects in superweight elements
Starting with element 104 (rutherfordium), new elements higher than actinides were first synthesized in the 1960s. These elements are unstable, and the longest decay half-life is only a few seconds (Rf251 half-life is 78 seconds). But rapid analysis techniques can allow people to study the chemical properties of these artificial elements.
Theory predicts that the outermost electron sublayer of these elements consists of 6d electron orbitals. This means that these super-actinides should have chemical properties similar to those of the transition group metal elements in the previous row of the periodic table: Rf should be like hafnium, element 105 (dubnium) like tantalum, and so on.
However, strong relativistic effects can weaken these cyclical properties. The chemical element "dubnium" (element 105) seems to be exactly this: its fluorinated complex is more similar to the fluoride of niobium than tantalum, and its other chemical properties are closer to protactinium. That is to say, it does not resemble a group 5 element at all, and its behavior is more like an extension of the actinide element.
There are also some indications that the Element Rf is also affected by relativistic effects: Rf tetrachloride is more volatile than the corresponding hafnium compound, and the trend prediction of the periodic table is the opposite.
Curiously, seaborgium (element 106) appears to be unaffected by relativistic effects and behaves just like the group 6 metals molybdenum and tungsten. Similarly, hassium (element 108) forms volatile tetroxides like osmium.
These studies push analytical techniques to the limits, including measuring only a few short-lived atoms.
Plate editing | Morgan