The award-winning CRISPR/Cas9 technology has become the most sought-after gene editing tool available today. Because it can mediate high-efficiency and high-precision genomic modification, it can realize the treatment of a variety of major diseases such as tumors, genetic diseases and infectious diseases, and shine in the field of life sciences. However, the lack of a suitable delivery system also limits the clinical application of CRISPR/Cas9 technology, so research in this area has also attracted considerable attention.
In the 12th issue of The Academic Online Live Broadcast, we invited Professor Ping Yuan, professor and doctoral supervisor of Zhejiang University, to share the application of gene editing non-viral vectors in the treatment of diseases.
Gene editing technology
Genome editing technology, which can precisely disrupt, insert, or replace DNA sequences at specific sites in the genome, plays a huge role in the study of gene function and the treatment of genetic diseases. Engineered endonuclease (EEN)-mediated gene editing technology has greatly improved the inefficiency of early gene targeting based on homologous recombination, allowing researchers to efficiently edit any gene of various cell types and organisms.
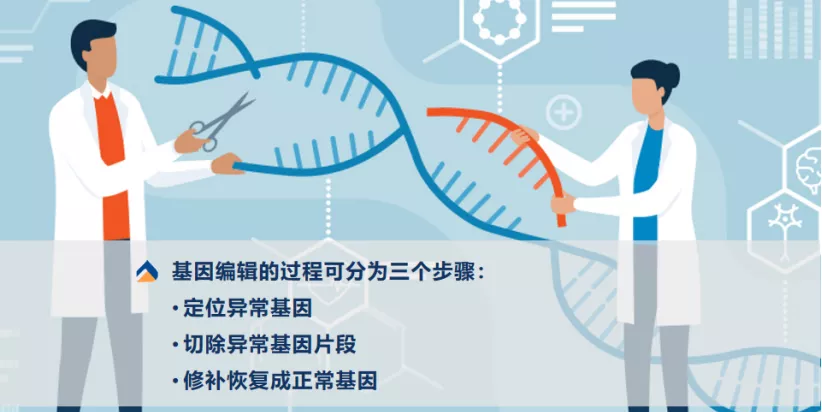
Figure | The three steps of the gene editing process
Currently, there are four types of instrumental enzymes, namely Meganuclease, zinc finger nucleases (ZFN), transcription activator-like effector nucleases (TALEN), clustered regularly spaced short palindromic repeats (CRISPR) and their associated proteins. It is most used in gene editing technology.
Whether it is ZFN, TALEN or CRISPR/Cas9, they are composed of two parts, the DNA recognition domain and the nucleic acid endonuclease, and are structurally similar. The ZFN technology has a zinc finger domain capable of identifying target DNA, while TALEN's DNA recognition region is the central domain of a typical tandem TALE repeat sequence, and the DNA shear region is a nucleic acid endonuclease domain called Fokl. The DNA recognition region of CRISPR is crRNA or guide RNA, and the Cas9 protein is responsible for the shearing of DNA. When the DNA binding domain recognizes the target DNA sequence, the nucleic acid endonuclease or Cas9 protein cuts the DNA, the target DNA double-strand breaks, and then initiates the DNA damage repair mechanism to achieve gene knockout and insertion.
Figure | ZFN, TALEN, CRISPR targeted dna cleavage DNA comparison
They also have similarities in the process of action. Specific DNA sites are identified and bound by the DNA recognition module, and then the specific sites are cleaved under the action of the relevant nucleic acid endonuclease, so that the insertion, deletion and gene fusion of specific sequences are completed with the help of the inherent HDR or NHEJ pathway repair process in the cell.
Figure | ZEN, TALEN, CRISPR differences comparison
By comparing many aspects with other technologies, we can see that CRISPR/Cas9 technology has both advantages and limitations. So what is the story of CRISPR/Cas9 technology's past and present lives?
Gene editing "magic scissors" - CRISPR
In 1987, Japanese microbiologist Ryojun Ishino first discovered CRISPR. Dr. Jennifer Doudna and Dr. Emmanuelle Charpentier, one of the founders of Scribe Therapeutics in 2012, used CRISPR to complete gene editing. Considered one of the most important discoveries of the 21st century, CRISPR was selected by Science as one of the "World's Top 10 Scientific Breakthroughs" three times in 2012, 2013 and 2015.
Clustered regularly interspaced short palindromic repeats sequences (CRISPR) are DNA repeats that provide bacteria and archaea with adaptive immunity to viruses and plasmids. CRISPR gene sequences are mainly composed of leader sequences, repeat sequences, and spacers. In 2002, Jansen's lab named the gene near CRISPR locus cas (CRISPR-associated) and discovered 4 cas genes (cas1, cas2, cas3, cas4).
The CRISPR/Cas system is a natural immune system of prokaryotes. When invaded by a virus, some bacteria are able to store a small portion of the virus's genes in a storage space called CRISPR in their DNA. When the virus invades again, the bacteria are able to identify the virus according to the fragments of the memory, cutting off the dna of the virus and making it ineffective.
CRISPR systems have type I, type II and type III., of which CRISPR/Cas9, as the most studied type II, is the third generation of gene editing technology that appears in addition to zinc finger nuclease (ZFN) and transcription activator-likeeffector nuclease (TALEN). Its mediated gene editing can be used to generate transgenic models, regulate transcription, regulate epigenetics, etc.
The CRISPR/Cas9 system is mainly composed of Cas9 proteins and single-stranded guide RNA (sgRNA), where cas9 proteins have the function of cutting DNA double strands, and sgRNA plays a guiding role. In the presence of protospacer adjacent motif (PAM) in the prototype spacer, the Cas9 protein can reach different target sites through base complementary pairing under the action of sgRNA guidance, and cut the target gene to achieve DOUBLE strand break (DSB).
3 key factors in CRISPR/Cas9 technical work
Guide RNA: RNA that locates part of the target gene. This was designed in the laboratory;
CRISPR-associated protein 9 (Cas9): "scissors" to cut off unwanted DNA fragments;
Donor DNA: The desired DNA fragment inserted after the original DNA strand has been cut.
Figure | SCHEMATIC OF CRISPR-CAS9-MEDIATED GENOME EDITING
There are two main mechanisms for DSB repair, namely non-homologous end-of-junction repair NHEJ and homologous recombinant repair HDR. Non-homologous end joining (NHEJ), NHEJ-mediated repair can produce inaccurate variable-length insertion and/or deletion mutations at DNA double-strand break sites. Other non-homologous recombination repair pathways also exist, such as microhomologymediatedend joining (MMEJ) and single-strand annealing pathways (SSA, Single-strand annealing).
The three pathways of non-homologous repair, NHEJ, MMEJ and SSA, are more distinct.
/ NHEJ pathway repair DSB damage is done by directly connecting the ends, which do not require homologous sequence recognition of large fragments, but only require 1-5 bp base pairing to repair, which is a type of error-prone repair.
/ The biggest difference between MMEJ repair and its approach to NHEJ repair is the difference in the length of the homologous sequence identified. The MMEJ repair pathway requires 5-25 homologous sequences of bases, which often results in the deletion of homologous fragments or gene rearrangement at the junction. This is also a bug fix.
/ Compared to NHEJ and MMEJ pathways, the SSA pathway requires a longer homologous sequence (>30bp) for identification. The SSA pathway can lead to genomic deletion, which is similar to the MMEJ pathway. In addition, SSAs, similar to the homologous recombination pathway, are also classified as homologous sequence-dependent DSB repair patterns, both of which require DNA clipping from 5' to 3' to produce 3' prominent single-stranded DNA.
Figure | Three pathways to non-homologous repair
Homology-directed repair (HDR), HDR-mediated precision repair can introduce precise point mutations or insertion mutations from single- or double-stranded DNA donor templates. The "homologous recombination" mechanism has important cellular biological significance in double-stranded DNA repair. DNA homologous recombinant repair is a complex signaling pathway involving multiple steps, with the key proteins BRCA1 and BRCA2. If a mutation in the BRCA gene causes the BRCA1 and BRCA2 proteins to lose function, it can cause homologous Recombination Deficiency (HRD) and MUTATIONS in RELATED GENES SUCH AS PALB2, CDK12, RAD51, CHEK2, ATM, or methylation of the promoter of the BRCA1 gene, resulting in genomic instability. When misidentification occurs in homologous recombination, performing "repairs" on non-template chromosomes can cause unpredictable harm to the cells. For example, the misidentification comes from a homolog of another parent, rather than a sister chromatid as a template to "repair" the damaged chromosome. The DNA sequences of maternal and paternal chromosomes are different in many locations, so this type of repair can transform the DNA sequence that needs to be repaired from the mother to the parent, or from the parent to the mother.
Figure | Homologous recombination often results in the exchange of genetic DNA sequences between chromosomal levels
Because CRISPR is simple, cheap, and efficient, it has become the most popular gene editing technology in the world, known as the "magic scissors" of editing genes. Scientists can efficiently and precisely alter, edit or replace genes in plants, animals and even humans, and the modified CRISPR technology can be widely used in biomedicine and other fields.
CRISPR technology has received significant repercussions in many areas of technical application and synonymous use, and there is a growing focus on safety, ethics and regulation.
CRISPR technology has broad application prospects and is expected to shine in medical, energy, agriculture, industrial and other fields
Develop new diagnostic tests, targeted drugs and treatments. By pairing CRISPR with other Cas proteins that can cleave the target DNA or RNA, and then cutting other molecules to produce visual signals, scientists can determine when a viral component is present. Scientists are using the technology to develop diagnostic tests to quickly identify diseases such as COVID-19. CRISPR/Cas9 is testing possible treatments for certain diseases, including sickle cell anemia and certain types of cancer. CRISPR can also be used to help control certain diseases by altering the properties of insects or other organisms that can transmit diseases. For example, scientists use CRISPR to make mosquitoes more resistant to malaria-causing parasites.
Development of biofuels. Gene editing can increase the yield of algal biofuels. Using CRISPR/Cas9 technology, scientists were able to find and remove genes that limit fat production. Synthetic Genomics, a U.S.-based biotechnology company that works on commercial genomics solutions to global energy and environmental problems, has created algae that produces twice as much fat, which is then used to produce biodiesel. To date, algae have not produced enough high levels of fat to support mass production of biodiesel. With CRISPR/Cas9 technology, the efficiency with which algae convert carbon dioxide into biofuels is expected to increase dramatically. Synthetic Genomics is currently working with oil company ExxonMobil. The partnership is expected to achieve the target of producing 10,000 barrels (oil units of measure equivalent to 120 - 159 liters per barrel) of algal biofuels per day by 2025.
Stronger, more disease-resistant crops. CRISPR can improve produce by increasing nutrient content, disease resistance, and survivability in harsh weather and soil conditions. In terms of traditional crop improvement, CRISPR technology can bring about increases in yield, quality, disease resistance and herbicide resistance. In agricultural breeding and accelerating crop domestication, CRISPR is innovating in combination with other technologies. Currently, CRISPR technology is widely used in model plants (Arabidopsis thaliana, tobacco) and some major crops, such as our common rice, wheat, corn, etc. CRISPR technology also facilitates plant genetic function studies and the selection of expected agronomic traits. For example, when researchers delete a small subset of specific cucumber genes, the plants are less vulnerable to viruses known to damage plants and cause severe crop losses. CRISPR gene editing inventors Professor Feng Zhang, Mr. David Liu and Mr. Keith Joung co-founded a new agricultural company called Pairwise. The company is committed to using gene-editing technology to harness the natural diversity of crops in new ways to address global food challenges.
More advanced industrial products. Various industries are exploring the potential uses of CRISPR. In addition to developing new biofuel accidents, bacteria that can rescue environmental disasters, as well as new materials, are also one of the main directions for industrial products to be used in conjunction with CRISPR technology. Caribou Bioscience, an innovative technology company focused on non-therapeutic applications of CRISPR, provides CRISPR-based tools to improve industrial fermentation processes and revolutionize the production of chemicals and enzymes. They also use CRISPR to manipulate microbes to produce new chemicals. Potential new biomaterials include fragrances, flavors and industrial cleaning products.
Figure | CRISPR technology application prospects opportunities and challenges coexist
CRISPR gene editing has also raised concerns about its safety, ethics and regulation
Security issues. Using CRISPR can have unintended consequences. For example, it can target "accidental" locations within DNA, producing changes that could lead to disease or other harm. Although some studies suggest that errors may be rare, they remain to be studied. In addition, CRISPR activity may compress cells and prevent editing. While some cells can recover after DNA changes, many cannot.
Ethical issues. Ethical questions include whether CRISPR will be used to enhance human traits, such as increased muscle mass, learning capacity, and memory, and whether all populations can be used equitably. Editing the impact of human reproduction using CRISPR also raises ethical questions. For example, the extent to which genetic changes affect future generations, whether research on human embryos should be allowed, etc.
Regulatory challenges. Many countries have struggled to decide how to regulate CRISPR and other gene-editing technologies. In the United States, for example, it is unclear whether gene-edited crops will be subject to the same regulations as conventional genetically modified (GM) organisms. Although the FDA began seeking public comment on its regulatory policies in 2017, new industry guidance on the technology has been delayed. In Europe, a 2018 court ruling determined that gene-edited crops would be subject to the same regulations as genetically modified plants. There are still many problems to be solved in the regulation of gene editing technology.
Viral vector
Viruses are a class of microorganisms that can effectively infect human cells and other animal cells. The ability of the virus is to efficiently introduce its own genes into the host cell, with good gene delivery efficiency. As a result, viruses have been engineered by scientists to be the primary delivery tool for gene therapy. Viral vectors most widely used in gene therapy include retroviruses (RV) and lentivirus (LV), adeno virus (Adv), and adeno associated virus (AAV).
Retroviral vectors are RNA viruses, but can be retrotranslated within contaminated cells to produce complementary strands of DNA, which can be used as a template to synthesize a second STRAND and a second STRAND that can be incorporated into the cellular genomic DNA. This virus can be transcribed and copied by the enzymes of the host cell, the RNA can synthesize proteins, repackage the virus, and the RNA is released from the cell and becomes an infectious virus, and the vector can be changed in different ways. Mediated processes allow the viral single-copy genome to enter the cell stably.
The genus lentivirus belongs to the retroviridae family in taxonomy and includes 8 viruses capable of infecting humans and vertebrates, and the primary infected cells are dominated by lymphatic and macrophages, resulting in the onset of infection in individuals. The lentiviral vector is a single-stranded RNA virus, and the more commonly used viral system is the four-plasmid system. Compared with other retroviruses, lentiviral vectors have the characteristics of a wider host, infectious capacity for both dividing and non-dividing cells, stable expression, and the ability to carry approximately 5 kbb and longer of the gene of interest after construction.
Adenovirus is a cellless linear double-stranded DNA virus that is currently divided into 5 genera in the adenoviridae family. In clinical and scientific research, Adv5 adenovirus vectors are primarily used. Adenoviral vector systems generally use human viruses as vectors and human cells as hosts, which are non-integrative viral vectors, so they provide an ideal environment for accurate post-translational processing and proper folding of human proteins. Adenovirus vectors also have the characteristics of a wide host range, low pathogenicity to humans, infection and expression of genes in proliferating and non-proliferating cells, high effective proliferation titers, no insertion mutagenicity, ability to amplify in suspension cultures, and simultaneous expression of multiple genes.
Adeno-associated viruses are a single-stranded, linear DNA-deficient type of virus that belongs to the microviridae family. Adeno-associated virus genomic DNA is smaller than 5 kb, has no envelope, and is a bare 20-sided particle. Adeno-associated viral vectors have the advantages of good safety, low immunogenicity, infection of dividing and non-dividing cells, and long-term stable expression of genes. Therefore, in in vivo and in vitro studies of the nervous system, adeno-associated viral vectors have attracted much attention.
At present, the viral vector is still the mainstream vector of clinical trials. The advantages of pathogenic carriers are high transfection efficiency, but disadvantages include specific populations can not be used, packaging capacity is limited, strong immunogenicity and lack of tissue targeting. Among them, the lentivirus host has a wide range, large gene capacity, high infection efficiency, can integrate genes into host cells, achieve long-term stable expression, and often build stable strains; adeno-associated viruses have low immunogenicity, a variety of serotypes, strong in vivo diffusion ability, and are commonly used for in vivo research; adenoviruses carry large exogenous gene fragments, high infection efficiency, mainly used for cells that are difficult to infect in vitro.
Figure | Comparison of viral vector technologies
Of course, there are many other types of viral vectors, such as vaccinia virus (VV), herpes simplex virus (HSV), bacteriophage vectors and so on. Taking HSV virus as an example, HSV virus has high titer, wide host range, large foreign gene capacity, and is specific for nerve cells, but its toxicity and immunogenicity need to be further verified. Therefore, HSA viral vectors are not widely used.
Non-viral vectors
The principle of use of non-viral vectors is to use the physicochemical properties of synthetic vector materials to mediate gene transfer. Compared with viral vectors, non-viral vectors have the advantages of high safety, large packaging size, weak immunogenicity, and controllable targeting, compared with viral vectors, non-viral vectors have lower costs and simpler preparations, which is convenient for large-scale production. In the expression of antisense oligonucleotides and other special exogenous gene fragments, viral vectors have an irreplaceable role of traditional viral vectors.
At present, the non-viral vectors under study mainly include liposomes, molecularly coupled receptors, polymers (poly-L-lysine, polyethyleneimide, etc.), composite carriers and nanoparticle carriers. Commonly used gene transfer liposomes include cationic, neutral and anionic liposomes, of which cationic liposomes are the most widely studied; and the inorganic nanoparticles used in gene transfer mainly include silicon, carbon nanotubes, iron oxides, etc., which mainly play a role in treating diseases by transporting drugs or biomolecules to organisms through cell membranes. Although non-viral vectors still have problems such as low transfection efficiency, they play an increasingly important role in the field of gene therapy.
Figure | Characteristics of non-viral vectors
Take lipid-based, lipid-like non-viral vectors as an example. Cationic lipids and lipid-like carriers can be loaded with nucleic acids by means of electrostatic interactions. At present, such non-viral vectors microRNA, siRNA and shRNA have been widely used in the delivery. Similarly, they can deliver CRISPR/Cas9 systems based on plasmid DNA, mRNA, and RNP. Many novel and high-performance lipid and lipid-like materials are designed for carrier construction, which often require PEG modification. Lipid vectors can be accumulated in tumor tissues through passive targeting, and ligand modification or structural modification of lipids can enhance the targeting of the vector, promote cell uptake, and improve delivery efficiency. After shielding the positive charge of the carrier and reducing the adsorption of protein, the circulation stability of such a carrier is also improved, and it can better meet the requirements of human administration.
Figure | Non-viral vectors for delivery CRISPR/Cas9 systems
Polymer polymers are formed by polymerization of short chain repeating units. The highly controllable structure of the repeating unit allows the polymer to be precisely designed according to the responsiveness requirements of the substances it contains. Structural diversity and functional versatility make polymers more likely to deliver pDNA.
Nanocarriers commonly used to deliver proteins include colloidal nanoparticles (such as liposomes and solid lipid nanoparticles), polymer nanomaterials (such as polymer films, nanocapsules, and micelles), inorganic nanocarriers (such as carbon nanotubes, quantum dots, mesoporous silicon dioxide, magnetic nanoparticles, gold nanoparticles, metal-organic frameworks, black phosphorus nanosheets) and the like. Magnetic nanoparticles can be used as contrast agents for magnetic resonance imaging; gold nanoparticles can produce photothermal effects; quantum dot particles can be traced for imaging; and some nanoparticles can carry drugs and genes at the same time. Such non-viral vectors can not only effectively deliver gene drugs into cells, but also achieve optimal anti-cancer effects through external interference.
Although the advantages of non-viral vectors are obvious, there are still many problems in the delivery process of non-viral vectors in the CRISPR/Cas9 system, such as how to be exempt from the recognition and clearance of the reticuloendothelial system (RES), how to achieve the enrichment of the nanoparticles at a specific location, and how to cross the hydrophobic cell membrane composed of phospholipid bilayers.
Soluble microneedling assists gene therapy, and the delivery of glucocorticoids presents a new path
Professor Ping Yuan's team at the School of Pharmacy of Zhejiang University used Cas9 ribonucleoprotein (RNP) gene editing technology that targets NLRP3 with high specificity and effectively treated inflammatory skin diseases (ISDs) with efficient percutaneous microneedle administration of glucocorticoids, a study titled "Microneedle-assisted genome editing: A transdermal strategy of targeting NLRP3 by." CRISPR-Cas9 for synergistic therapy of inflammatory skin disorders" paper was published in Science Advances, a science sub-journal. This research result has brought soluble microneedle patch gene editing therapy into people's field of vision.
Inflammatory skin diseases (ISDs) are one of the most stubborn diseases and are often characterized by the activation of innate and adaptive immune responses by producing pro-inflammatory cytokines. Inflammatory skin diseases such as psoriasis and specific dermatitis (AD) are becoming increasingly prevalent and are one of the major threats to public health. At present, patients can only choose a treatment regimen from a small number of drugs, and although glucocorticoids and immunosuppressants are first-line treatment options, most patients develop resistance to glucocorticoids after long-term treatment, and the therapeutic effect is limited. Therefore, the development of alternative treatment options is an important breakthrough point for providing medical options for patients who are resistant to glucocorticoids.
Most studies have shown a strong correlation between NLRP3 activation and glucocorticoid resistance, and although there are many studies involving small molecule inhibitors that interfere with the activation of the upstream correlators of NLRP3 to indirectly achieve therapeutic purposes, the content of drugs that can reach the subcutaneous layer after oral administration is very limited and does not achieve efficient therapeutic purposes. Therefore, improving safety and effectiveness has become a top priority in this R&D direction.
Professor Hirabachi's team developed a soluble microneedle (MN) patch encapsulated in microneedles with a nanocomposite of Cas9 ribonucleoprotein (RNP) that targets NLRP3 and a nanocomposite containing dexamethasone (Dex). When inserted into the skin, microneedles can quickly dissolve and release two nanocomplices, which are subsequently internalized by cuticle cells and surrounding immune cells, effectively treating inflammation in the subcutaneous layer. Microneedles transdermally deliver Cas9 RNPs and Dex nanocomposites, destroying subcutaneous intracellular NLRP3 inflammatory bodies, and are effective in the treatment of specific dermatitis (AD) and psoriasis under synergistic therapy with Dex.
When screening sgRNAs sequences, it was found that NLRP3 gene loci were edited in DC2.4 and 3T3 cells mediated by CMAX, a commercial transfection reagent, with Indel efficiencies of up to 35.4% and 32.5%, and was also confirmed by Sanger sequencing. In addition, the experiment found that the Indel efficiency of the NLRP3 genome site increased from 29.6% to 36.2% after adding PLGA/Dex nanocomplexes to DC2.4 cells. In 3T3 cells, Indel efficiency also increased from 19.1 to 31.7%. The results confirmed that the PLGA in the Dex nanocomposite may expand the nuclear pores, promote cas9 nucleation, and improve the editing activity of NLRP3 gene loci.
Microneedle-mediated, graded transdermal therapy strategies maximize the therapeutic effects of gene editing and glucocorticoid synergistic therapy. This approach not only has great potential in the treatment of inflammatory skin diseases, but also provides new ideas for the combination of percutaneous drug delivery and gene editing therapy.
At present, most of the research in the field of non-viral vector gene therapy is still carried out in vitro, and the results in vitro and in vitro are different. Therefore, the future development of non-viral vector gene therapy will be more relevant to in vivo research.
Representatives of non-viral products that are currently on the market
Neovasculogen
The first non-viral gene therapy product, launched in 2012 by the Human Stem Cells Institute in Russia; consists of a plasmid vector encoding vascular endothelial growth factor (VEGF-165); indications: treatment of severe acromembria.
Collategene
Launched by AnGes Corporation of Japan, it was approved for use in Japan National Health Insurance in 2019. Collategene consists of pVAX1-HGF, an isomer that expresses HGF728 in humans. Its main indications are the treatment of arteriosclerosis obliterans and thromboangiitis obliterans.
Spinraza (Nusinersen)
Biogen and Ionis Pharmaceuticals in the United States jointly developed and were approved by the FDA in 2017. It alters the splicing process of the RNA by binding to mRNA transcribed from the SMN2 gene, thereby increasing the expression of normal SMN proteins. Therefore, this therapy can increase the level of normal SMN protein in patients with SMA who are inactivated by the SMN1 gene, thereby maintaining the survival of motor neurons. Its main indication is the treatment of spinal muscular atrophy in children and adults.
Delivery of CRISPR/Cas9 for various applications has been implemented both non-viral and viral vectors. Gene therapy has also shifted from basic research to drug development and industrial production. Gene therapy has sufficient market space in the treatment of blood tumors and rare diseases, and the future can be expected. With the improvement of research and development level and the improvement of preparation conditions, the combination of scientific research and clinical needs and the continuous improvement of the transformation process, gene therapy will eventually enter the homes of ordinary people in the future with the support of insurance payments.
<h1 class="pgc-h-arrow-right" data-track="148" > about the cluster</h1>
Zhitu is a scientific and technological big data platform integrating scientific and technological data, intelligent analysis and academic services, with the mission of perspective on the future nature of science and technology, providing research databases, analytical tools, academic conferences, academic communities and other functions for scholars, universities, scientific research institutions and relevant groups engaged in the transformation of scientific research achievements, and effectively amplifying the influence of academic research through the media dissemination of original research.