Abstract As one of the frontier fields of condensed matter physics, superconductivity has flourished for more than 100 years, and related research has contributed to at least 5 Nobel Prizes in Physics, with at least 10 winners. With the continuous development of superconductivity research, the contributions of Chinese scientists in it are becoming more and more important, especially in the field of iron-based superconductivity, which has led the world. This article will introduce the research of superconductivity and its importance from the experiences of Nobel laureates, and explore the possible Nobel Prizes in the field of superconductivity in the future.
Every year in early October, the scientific community is buzzing because it's time for the Nobel Prize to be announced. Although the Nobel Prize does not represent the highest level of academic research, it has been subtly become the highest honor in the scientific community. Since the first Nobel Prize in Physics was awarded to Roentgen in 1901, more than 200 scientists have been awarded the Nobel Prize in Physics in more than a hundred years. In the history of the Nobel Prize in Physics, the four major fields of astrophysics, particle physics, atomic molecules and light physics, and condensed matter physics have been rotated in turn, and there are about 50 Nobel Prizes related to condensed matter physics. Of these scientists, at least 10 (Figure 1) were awarded the Nobel Prize in Physics directly for their research on superconductivity. They were Kamerin Onnis (1913), John Bardeen, Leon Cooper, John Schlieffer (1972), Ival Jaevo, Brian Josephson (1973), George Bernoz, Alexander Müller (1987), Alexei Abrikosov, and Vitaly Günzburg (2003).
To be precise, superconductivity is only one branch in the field of condensed matter physics, and it is a branch of the entire branch of physics. Why is it that a small field of superconductivity has such a strong vitality and is so favored by the Nobel Prize Committee? Superconductivity, literally, means "superconductivity". Materials in the superconducting state have zero resistivity and have very strong full antimagnetism, that is, their internal magnetic induction intensity is also zero when they are in the external magnetic field. Such superior electromagnetic properties are unmatched by any other material. Therefore, wherever electricity or magnetism is needed, superconductivity has the opportunity to show great magic. For example, the use of superconducting materials to replace traditional copper-aluminum alloy transmission lines can save 15% of the transmission losses that are still difficult to avoid in high-voltage transmission. The superconducting magnet made of superconducting coil can achieve a magnetic field strength of more than 25 T, and with conventional magnets, it can even achieve a super stable magnetic field of more than 45 T. Nowadays, most of the hospital MRI images use superconducting magnet technology, and the highest resolution is enough to map all 86 billion neurons in the human brain! Using the powerful electromagnetic characteristics of superconductivity, a high-speed stable superconducting maglev train of 604 km/h can be realized, and the journey from Beijing to Shanghai can be shortened to about 2 h. The quantum properties of superconducting materials have more enviable application potential, because the formation of superconductivity is precisely due to the pairing of electronic systems in the material to form a macroscopic quantum condensed state of electron collectives. Using the quantum interference behavior between superconducting electrons collectively, a superconducting quantum interferometer that reaches the quantum limit with the highest precision can be realized. The superconducting qubit, a logical operation unit made of superconducting quantum effects, can construct a superconducting quantum computer with a computing power hundreds of thousands of times higher than that of a conventional computer. Even if it is a simple use of superconducting material pairs
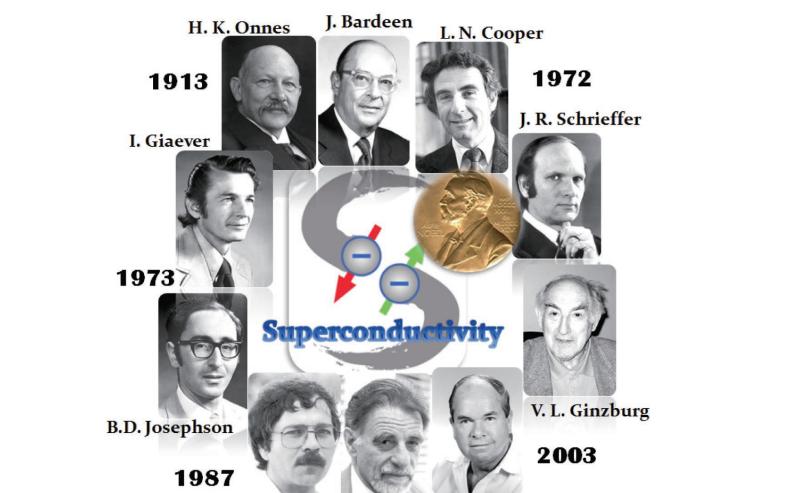
Figure 1 10 scientists who won the Nobel Prize in Physics for superconductivity
The sensitive characteristics of light, electricity, and magnetism can also be made into extremely sensitive single-photon detectors, which are the key components of the current quantum satellite communication technology; superconducting filters with excellent signal-to-noise ratios can also be made to provide superior communication quality assurance in the era of information explosion; and superconducting terahertz generators and receivers can also be made, which have great potential in the military and security fields. The above examples are only a small part of the field of superconducting applications. It is precisely because superconductivity is so alluring that it has driven generations of scientists to be fascinated.
The importance of superconductivity is far from being limited to its vast application potential. In fact, the study of superconductivity has led to the development of condensed matter physics and even physics as a whole. In experiments, the study of various complex properties of superconducting materials has prompted people to continuously improve the accuracy of various measurement techniques, improve the measurement environment index, and develop new measurement methods. For example, the photoelectron spectroscopy technology for the microscopic state measurement of material electrons, its energy resolution from the initial 100 meV, to the current 1 meV or less, solid state magnetic resonance technology can reach a low temperature of 10 mK, resistance measurement technology can reach 2 million atmospheres of ultra-high pressure, the new resonance non-elastic X-ray technology has been rapidly developed. The development of these experimental technologies is the hardware basis for the continuous emergence of new phenomena and discoveries in condensed matter physics in the past hundred years. Theoretically, the idea of electron pairing and the concept of spontaneous symmetry developed by the study of superconductivity have affected cold atoms, particle physics, cosmology, and other fields; and the understanding of associative electron effects has advanced people's understanding of multibody interactions, and instead adopted topological order to re-describe our microscopic world. New theoretical frameworks are taking root, the edifice of condensed matter physics is about to be rejuvenated, and even the whole of physics may be transformed again. It is precisely because superconductivity is so attractive and important that it has been a research content at the forefront of physics for more than a hundred years and has been valued by the Nobel Prize Committee. These stories about superconductivity may be traced from the history of the Nobel Prize, and the scientists who won the award for superconductivity are each an interesting legend. In 1913, Kamerin Onnis was awarded the Nobel Prize in Physics for his research on the physical properties of cryogenics and the successful preparation of liquid helium. Among them, the study of cryogenic physical properties includes the first superconductor he discovered in 1911- metallic mercury. The key to the discovery of metal mercury superconductivity is to obtain liquid helium to provide a low temperature environment. According to the ideal gas state equation, pressurizing various gases can become liquids, and the boiling point of the corresponding liquefied gases is very low at atmospheric pressure. For example, the boiling point of nitrogen under atmospheric pressure is 77 K, and further decompression cooling can reach a low temperature environment of about 40 K. After the other gases were conquered, only hydrogen and helium, the two lightest gases, remained unliquefed. Onnis built a cryogenic physics laboratory at leiden University in the Netherlands, the main purpose of which was to overcome the liquefaction of hydrogen and helium. Guided by his friend Van der Vals (who won the Nobel Prize in Physics in 1910), Onis realized that the ideal gas equation of state was not enough, it was necessary to consider the van der Wals equation for the interaction between gas molecules, and soon conquered the liquid hydrogen technology. Finally, on July 10, 1908, Onnis liquefies helium (Figure 2) to obtain a liquid helium with a boiling point of 4.2 K at atmospheric pressure. With liquid helium to further reduce the pressing cold, a low temperature of about 1.5 K can be achieved, while He-3 refrigeration can reach a low temperature of less than 0.1 K. Onnis's success opened the door to cryogenic physics. Prior to this, the conductivity of metals at low temperatures was not understood, because the experimental conditions at that time could not reach a low enough temperature. It has been speculated that the resistivity of metals may rapidly increase to divergence at low temperatures, or decrease to a certain extent to stay on the residual resistivity value caused by impurities and defects. Onnis has always believed that if you measure extremely pure metal materials, it is possible that the resistivity will continue to decrease to zero when it tends to absolute zero. With liquid helium as a low-temperature weapon, Onnis quickly measured the low-temperature resistivity of various metals and found that platinum and gold, which have the best electrical conductivity at room temperature, have residual resistivity at low temperatures. Finally, in 1911, Onnis asked laboratory assistants to start measuring the low temperature resistivity of metal mercury, mainly because mercury can be distilled and purified, and the purity can be extremely high, which is called the perfect metal. A magical day began on an ordinary weekend on April 8, when a lab assistant was measuring the low temperature resistance of mercury and found that the resistance was suddenly undetectable when it crossed 4.2 K, that is, the reading was 10-5 Ω below the resolution of the instrument. When Onnis heard about this, he immediately asked his assistants to repeat the experiment and recorded the words "super conductive" in his notebook. But because 4.2 K coincides with the boiling point of liquid helium, one wonders if there is a problem with the measurement. It took Onnis and his colleagues several more months to confirm this phenomenon before they carefully published the relevant results in the journal of Leiden University in the Netherlands, which they named "superconductivity." Proving whether the resistivity of the superconductor is zero is actually a very tricky thing. Technicians at the University of Leiden's Cryogenic Physics Laboratory, even 16 years after Onnis's death, are repeating relevant experiments to continuously improve their experimental precision. In the end, it was shown that the resistivity of the superconductor was much smaller than that of the conventional metal platinum with the lowest resistivity at room temperature by 10 orders of magnitude. If a steady current of 1 A is achieved in the superconducting ring, then it can be continuously and stably guaranteed that it will not decay for 100 billion years, longer than the age of the universe! In this case, people can completely think that the resistivity of the superconductor is perfect zero, which makes people sigh at its magic!
Figure 2 Monument of leiden University to on onys' acquisition of liquid helium
In 1972, after nearly 60 years, superconductivity won the Nobel Prize again. The three winners, John Bardeen, Leo Cooper, and John Schliefer, were awarded "for their commonly developed theory of superconductivity, commonly referred to as BCS theory." This is an intriguing history, indirectly due to the fact that the Nobel Prizes of the 1920s and 1950s were awarded mainly to quantum mechanics, particle physics and astrophysics, but more directly because the field of superconductivity was not hot enough at that time. After onnis discovered the first superconductors, many metal elements were successively proved to be superconductors, and superconductors were also found in many metal alloys, but it was depressing that their critical temperatures were extremely low, almost all below 20 K (Figure 3). Such a low temperature means that liquid helium must be relied upon to maintain low temperatures, but helium is a noble gas, and liquid helium is difficult to produce, so the cost of superconducting applications is very high. Another important issue is that the understanding of the microscopic nature of superconductivity has been at a very difficult stage. Many extremely intelligent scientists, such as Einstein, Feynman, Heisenberg, and others, have tried to establish a microscopic theory of superconductivity, but they have failed. It can be said that the theoretical problem of superconductivity has been put into a large group of Nobel Prize winners, but it is still fruitless. In the 1950s, quantum mechanics has matured, and the solid physical theory based on the framework of quantum mechanics has also developed, and people have gradually gained a deep understanding of the microscopic conductive mechanism in solid materials. After completing his research on semiconductor transistors, Bardeen was acutely aware that superconductivity would be the next opportunity for a breakthrough. So he pulled up the postdoc Cooper and the graduate student Schriver, formed a "three-person group" combining old, middle and young, and finally made a breakthrough in 1956 after a few months of concentrated efforts. Cooper first proved that an electron pair could be stable if there was a weak attraction interaction between electrons in a metal; Schriver then found a wave function that appropriately described the pair of electrons and gave the equation of motion of the superconducting electron; Bardeen pointed out from the leader's point of view that the electrons interacted weakly by exchanging atomic vibration quanta, and led Cooper and Schriver to theoretically prove the existence of a zero resistance effect and complete antimagnetism, hence the name of the three of them The word is named BCS Theory. The microscopic theory of superconductivity almost perfectly explains the superconducting properties of conventional metals, in which the idea of how electrons produce attraction interactions has far-reaching effects.
Figure 3 The age of discovery of various representative superconducting materials and their critical temperature Figure 3 The age of the discovery of various representative superconducting materials and their critical temperature
Here are a few interesting stories. When Bardin asked Schriver to do research in this direction, he asked him how many grades he was a graduate student, and he replied that he was newly enrolled, and then Bardeen said, "That line, let's do the problem of superconductivity first, and it will be okay to delay you for a few years, and you can find other topics and then find a way to graduate." Schlieffer was also extremely painful in his search for a superconducting wave function, but he was a man of broad interests, including frequently listening to lectures on particle physics across disciplines. On a train back to school on a vacation, Schriver was finally inspired by a formula in particle physics to write down a seemingly unconventional superconducting wave function that turned out to be correct. He was only 24 years old at the time. Bardeen himself is a legend in the field of physics, the only scientist in history to have won the Nobel Prize in Physics twice (Fig. 4). His first Nobel Prize came in 1956, when he had just formed the "Superconducting Dream Team", because he and Bell Labs Shockley, Bratton and other three people (Figure 5) invented the semiconductor transistor, which became the cornerstone of modern semiconductor and computer technology. When he won the first Nobel Prize, the presenter asked Bardeen if he had brought his family with him, and he said that he had brought two sons, but let them stay in the hotel, and did not expect to come to the Nobel Prize site, so the award presenter half-jokingly said: "Then next time!" It turned out that there was a next time, and this time in 1972, Bardeen decisively took his sons to accept the edification of the Nobel Prize. They later became famous scientists. Badin visited China twice in 1975 and 1980, and in his report at the Institute of Physics of the Chinese Academy of Sciences, he explained the secret of success: effort, opportunity, and cooperation. Also here, during this time period,
Figure 4 Bardeen's Nobel Prize certificate and two medals Figure 4 Badin's Nobel Prize certificate and two medals
China has set up the first vanguard of superconductivity research and has achieved a number of world-cutting results in subsequent superconductivity research. These scientific pioneers have now blossomed and cultivated a number of the world's main forces in superconducting research.
Figure 5 Bardeen, Bratton, and Shockley at Bell Company
Then, in 1973, Superconductivity won the Nobel Prize in Physics again, this time for the applied research of superconductivity. Brian Josephson shared the Nobel Prize in Physics with another Jiang Qiling yu Nain Semiconductor Tunneling Junction for his theoretical prediction of the superconducting tunneling effect (later known as the "Josephson effect"), Iwar Jaevo, for the experimental realization of superconducting tunneling knots. Josephson was only 33 years old when he won the Nobel Prize, second only to Lawrence Bragg (25) and Lee Jung-doo (31), and he was only 22 years old when he did related research work, and he was still in the second year of graduate school. When Josephson was just a graduate student, his supervisor was Pipard, one of the superconducting cattle at the time, and he asked Josephson to find his own research topics. By listening to a speech by another bull physicist at the time, Philip Anderson (1977 Nobel Laureate in Physics), Josephson felt that there could be a collective quantum tunneling effect between two superconductors. He tried to do the calculation using a simple variational method, and showed the preliminary results to the tutor, and the result was a big criticism. Josephson was not convinced, and went to send the calculation results to Bardin ( who had become famous for BCS theory at the time ) , and again drew a sharp rebuke , and Bardeen said that he did not believe in such a strange result. Fortunately, Anderson expressed his support for the young Josephson and encouraged him to write a paper for publication, and he finally managed to finish his career. Due to the opposition of Bardeen and his mentors, Josephson was a little discouraged by scientific research after graduation. However, Anderson noticed that Jaevo had actually observed the tunneling current between the superconductors 2 years earlier, and had his colleagues repeat similar experiments and successfully observe the results predicted by Josephson's theory. It is believed that there can be a quantum collective tunneling effect between superconductors, and that the tunnel current is extremely sensitive to magnetic fields. Bardeen also took a 180° turn in joseph's attitude after the results of the experiment, and then tried to support his scientific career, which may have led to his successful Nobel Prize. Regrettably, industry acknowledgement may have come a little late to Josephson, and his research interests quickly shifted from physics to biology. Especially in his later years, Josephson did a lot of "scientific research" on some supernatural phenomena such as the specific functions of the human body, and he himself was fascinated by them, and even gave lectures on related topics at a famous university in China, so he gradually faded out of the orthodox scientific field. The Josephson effect is the basis for the application of superconducting electronics, and superconducting quantum interferometers, superconducting qubits, and superconducting quantum computers all rely on this effect. In addition to the strong electrical applications of superconductivity, this opens up another half of the world for superconducting applications - weak current applications. In particular, the development of superconducting quantum computers has been very rapid in recent years, and China has invested in the construction of Alibaba's quantum computing laboratory in Shanghai, looking forward to building a subcomputer produced abroad within 10 years. In 1987, the next Nobel Prize for superconductivity was awarded to the study of superconducting materials, the discovery of high-temperature superconductors, by two IBM engineers, Bernards and Müller. People should not be surprised by their work units, because the large companies of the time had basic scientific research departments, and there was no difference between them and today's national laboratories, and Bell Labs produced a group of famous scientists like Bardeen. Bernoz is Müller's Ph.D., and after graduation, he stayed at the company to continue his research with his supervisors, with the aim of finding superconductivity from oxide ceramic materials. Their exploration is not supported by everyone, because almost all oxide ceramic materials are insulators, let alone superconductivity, and even conduct electricity are difficult. However, they did not give up, "we never thought we would be successful, we could only keep a low profile, work overtime and overtime, borrow colleagues' equipment to complete experiments." 」 20 years later, Bernoz recalled. Finally, in 1986, they noticed that French scientists mentioned that a rare earth copper oxide La-Ba-Cu-O system has metal conductivity, and then they quickly synthesized the material, and measured the resistance to a low temperature, and found that the resistance below 35 K dropped to zero. Because only one of the three samples has a zero resistance effect, they also carefully used the term "possible high-temperature superconductivity" when publishing their papers. After the experimental test of the antimagnetism was continued in the following year, it was determined that it was superconductivity. The critical temperature of 35 K seems to be not high, but at that time, the critical temperature record of 23.2 K maintained by the superconducting material Nb3Ge has been broken, compared with the previous low temperature superconductivity that was always below 20 K, which is already considered "high temperature", so it is called "high temperature superconductor" (Figure 6). Bernards and Müller's paper was published in June 1986 and won the Nobel Prize in Physics in October 1987, with a gap of 16 months, almost setting a record for the fastest Nobel Prize to work. Faster than them were two Chinese, Yang Zhenning and Lee Jeong-do (who were still holding republic of China passports at the time of the award), who won the Nobel Prize in 1957 for their theoretical work on the metaphor of weak interaction.
Figure 6 Bernoz shows the structure of a La-Ba-Cu-O high-temperature superconductor
Why Bernards and Müller were able to win the Nobel Prize so quickly is also due to the contributions of Chinese/Chinese scientists. After the work of Bonoz and Müller, Zhao Zhongxian's team at the Institute of Physics of the Chinese Academy of Sciences (Figure 7) and Zhu Jingwu of the University of Houston and Wu Maokun of the University of Alabama were very excited about this, because the newly found "high temperature superconductivity" material properties are very compatible with their long-term exploration of high-critical temperature superconductors. In just the past few months, these two superconducting exploration teams from China and the United States have not only completely independently repeated the work of Bernards and Müller, but also found signs of higher superconducting temperatures, and finally in February 1987, they independently achieved superconductivity of 93 K in the Y-Ba-Cu-O system, which led to a round of scientific and technological competition to brush the critical temperature of superconductivity. From 35 K to 90 K, this amazing leap illustrates the superiority of copper oxide superconducting materials, which means that it can fully enter the liquid nitrogen temperature zone above 77 K, replacing the extremely expensive liquid helium to maintain a low temperature environment, making large-scale applications possible. In addition, according to BCS theory, the superconducting critical temperature of metals or metal composites cannot exceed 40 K, which is called the McMillan limit. Superconductivity in copper oxides clearly breaks through the limits of the theory, and also shows that the BCS theory itself has limitations. Generally speaking, superconductors that are believed to be able to break through the critical temperature above 40 K are called "high-temperature superconductors" (Note: It is also said that it is 20 K), and cannot be called "unconventional superconductors" by the superconductors described by BCS theory. Copper oxides belong to both, and there are some superconductors whose critical temperatures are not high, but they also belong to unconventional superconductors. The discovery of high-temperature superconductivity was extremely violent at that time, and the March meeting of the American Physical Society in March 1987 specially set up a "Symposium on High Critical Temperature Superconductors." More than 3,000 physicists from all over the world crammed the 1,100-person reporting hall, and the frenetic meeting discussion lasted seven hours until 2 a.m. That conference, known as the "Rock And Roll Music Festival in Physics," was an epoch-making milestone in the history of superconductivity research. Although there is speculation as to why no Chinese/Chinese scientists shared the 1987 Nobel Prize in Physics, the most immediate reason is that their results were released after the Deadline for Nominations for the Nobel Prize on January 31, 1987. What is admirable is that under the circumstance that material conditions and experimental conditions were extremely scarce at that time, it was not easy for Chinese scientists to independently make such important scientific contributions to the world's frontiers.
Figure 7 China's high-temperature superconductivity exploration team led by Zhao Zhongxian
The most recent Nobel Prize for superconductivity was received in 2003 by Alexei Abrikosov and Vitaly Günzburg for their pioneering theoretical work in superconductivity, and in the same year, Anthony Leggett in superflow theory. This is the second Nobel Prize for superconductivity theory research. The theories of Abrixov and Ginzburg differ from the BCS theory, which is based on a microscopic theory of quantum mechanics, while the former is only a so-called "idealistic theory". It is necessary to mention here another famous Soviet theoretical physicist, Lev Landau, who was the founder of the Soviet physics community and made the most outstanding contributions to the theory of condensed matter physics, and he was only matched by Fermi, who together constructed the cornerstone of condensed matter physics - landau-Fermi liquid theory. It is precisely landau that invented the "landau phase transition theory" based on phase transitions and critical phenomena, which can describe not only superconductivity phenomena, but also a series of phase transition behaviors in condensed matter physics such as supercurrent phenomena. However, Landau's theory presupposes an imaginary "order parameter" without regard to the specific physical microscopic mechanisms in the material, so it is called "image theory" ( ) . It was together with Landau that Günzburg developed the superconductivity theory of superconductivity--called the "Günzberg-Landau theory." However, the theoretical equation of light does not explain the problem, and Abrikosov exerted his mathematical talent to give an analytical solution to this theoretical equation mathematically, and divided the superconductors into two categories: the first type of superconductors has only one critical magnetic field, above which is the positive normal with resistance, and below it is the superconducting state of zero resistance; the second type of superconductor has two critical magnetic fields, above the upper critical field is a positive normal with resistance and no antimagnetism, below the lower critical field is a superconducting state with zero resistance and complete antimagnetism, and the middle is It is a mixture of zero resistance but not full magnetic resistance. In particular, Abrikosov pointed out that in the mixed state of the second type of superconductor, the magnetic field can enter the superconductor in the form of quantized magnetic flux vortexes, and the magnetic flux corresponding to each magnetic flux vortex is a quantized minimum magnetic flux unit - "quantum flux", and they will form an orderly arrangement of magnetic flux lattice. This theoretical prediction was then directly observed experimentally, proving that superconductivity is a quantum effect. The existence of superconducting magnetic flux quanta means that superconductors are very complex and changeable in many cases, which not only brings many difficulties to the strong electrical application of superconductivity, but also brings many opportunities to the weak electrical application of superconductivity. Because of the long-standing difficulties in superconductivity applications, Abrikosov's work has not been taken seriously by the Nobel Prize Committee. In 1962, Landau won the Nobel Prize early for other condensed matter theories such as liquid helium, or it may be that the committee was worried that the genius who suffered a car accident would not survive for many years, and quickly issued the prize but regretted it. When he won the Nobel Prize in 2003, Ginzburg was 87 years old and Abikosov was 75 years old (Figure 8). Soon after, Günzburg died in 2009, and Abikosov died in 2017. Indeed, to win the Nobel Prize, health and longevity are also one of the important prerequisites.
Figure 8 Nobel Prize certificate from Abikosov
The above 10 Nobel laureates directly related to superconductivity are also incomplete statistics. In fact, the study of superconductivity and superflow theory is in the same vein, and many physics journals have classified it into one category. The idea of electron pairing in superconducting BCS theory and the concept of symmetry breaking are widely used in the fields of superflow and particle physics, such as Yukawa interaction and Higgs mechanism. Although the Nobel Prizes won by Leggett, Landau, and others were awarded to them for their supercurrent theoretical work, they were more or less related to superconductivity. Hideki Yukawa, Yoichiro Minami, and Higgs also won the Nobel Prize in Physics. In 2016, the Nobel Prize in Physics was awarded to three scientists, David Solis, Duncan Haldane, and Michael Kostlitz (Figure 9), to illustrate their theoretical discovery of topological phase transitions and topological matters. Among them, Kostritz and Solis proposed a theoretical model of phase transition when studying supercurrent and superconducting phenomena, which is called "KT phase transition"[1]. In recent years, the study of the topological properties of materials has opened a new door to condensed matter physics, and some "ghost particles" or "angel particles" that have been difficult to find for many years in the field of particle physics have also been discovered in condensed matter matter, and physics is also brewing a change. What is certain is that there will also be a number of Nobel Prizes in the field of superconductivity. So, what research will the next Nobel Prize for superconductivity award be awarded? Awarded to scientists? To which scientists?
Figure 9 Three Nobel laureates in physics in 2016
Hideo Nishino (Figure 10), a Japanese scientist who discovered iron-based superconducting materials in 2008, is one of the "candidates" for the Nobel Prize for Superconductivity, and in recent years has been predicted by many institutions to win the Nobel Prize. Iron-based superconducting materials are the second largest high-temperature superconducting family discovered after copper oxide high-temperature superconductors, with a very large material system, which is worthy of being the star of new superconducting materials. Interestingly, when Hideo Nishino discovered the iron-based superconductor, the highest critical temperature was 26 K in the La-FeAs-O-F system, which did not exceed the McMillan limit of 40 K, and it could not be confirmed that it was a high-temperature superconductor. It was scientists from China who achieved this major breakthrough and raised the superconductivity temperature of the block to 55 K. What is even more valuable is that Chinese scientists have rapidly grown from followers in past superconductivity research to a wave leader in this new wave of iron-based superconductivity. Not only has a number of breakthrough discoveries been made in the exploration of iron-based superconducting materials, but also a number of world-leading achievements have been obtained in the experimental research of iron-based superconductivity, and it has taken the lead in iron-based superconductivity theory
Figure 10 Hideo Nishino, discoverer of iron-based superconductivity Figure 10 Hideo Nishino, discoverer of iron-based superconductivity
He has put forward various theoretical explanations and models, and successfully led the world in the application of iron-based superconducting materials in strong electricity, occupying the forefront of the world in almost all aspects. In a quote from Science magazine: "The new superconductor will push Chinese scientists to the forefront of the world." In 2008, iron-based superconductivity was rated as one of the world's top ten scientific advances by many institutions. The Chinese iron-based superconductivity research team (Figure 11) won the 2009 "Qiushi Outstanding Scientific Achievement Collective Award" and the 2013 National Natural Science First Prize; Chinese scientists Zhao Zhongxian and Chen Xianhui won the 2015 "Matias Award", the highest honor in the field of superconducting materials exploration for their outstanding contributions to iron-based superconducting materials; Academician Zhao Zhongxian, a leading scientist in China's high-temperature superconductivity research, won the 2016 national highest science and technology award; and Xue Qikun of Tsinghua University was awarded the single-layer iron selenium award Research such as superconductivity won the 2016 "Future Science Award" Material Science Award. This series of awards shows that Chinese scientists have occupied a place in the vanguard of the world's superconducting research.
Figure 11 China iron-based superconductivity research team
The hydrogen sulfide high-pressure superconductivity discovered in 2015 could also be a popular "candidate" for the next Nobel Prize for superconductivity. Two German scientists, Drozdoff and Ermez (Figure 12), successfully measured the resistance and susceptibility of H3S at 2 million atmospheres and found a superconductivity of up to 203 K, the highest recorded critical temperature for superconductivity so far. Although the microscopic mechanism of superconductivity in this system is relatively clear, and such high external pressure also means that the application is almost impossible, it is also an inspiring attempt to break records. In addition, in the exploration of superconducting materials, organic superconductors and heavy fermion superconductors discovered in 1979 belong to the other two families of unconventional superconductors and may also be the next Nobel Prize for superconductivity. Of course, people are more looking forward to the day in the future, the superconductor at room temperature of 300 K will be discovered, which will surely give birth to another superconductivity Nobel Prize.
Figure 12 Two German scientists Drozdorf and Ermez who discovered hydrogen sulfide superconductivity
Finally, the Nobel Prize in superconductivity research will surely be the microscopic theory of high-temperature superconductivity or the microscopic theory of unconventional superconductors. As mentioned earlier, copper oxide high-temperature superconductors and iron-based high-temperature superconductors can no longer be described by the traditional BCS theory, and the difficulties encountered there are even breakthroughs in the Landau-Fermi liquid theory. That is to say, once the microscopic theory of high-temperature superconductivity has made a substantial breakthrough, then the cornerstone of traditional condensed matter physics research may be reconstructed, and the impact on the entire physics is unprecedented. Of course, the Nobel Prize is 100% predictable, and without winning the Nobel Prize, scientists will continue to fight for the mystery of superconductivity. It is gratifying that in recent years, China's contingent on the road of superconductivity research has continued to grow and develop, and the contributions of Chinese scientists have become more and more important, more and more important, and more and more cutting-edge in the exploration of superconducting materials, the study of superconducting physical properties, the study of superconducting mechanisms, and the application of superconductivity technology. We have every reason to believe that the next major breakthrough in the field of superconductivity may occur in China, or even on our own side. At that time, whether it is necessary to use the Nobel Prize to weigh it is no longer important.