Happy New Year HAPPY NEW YEAR
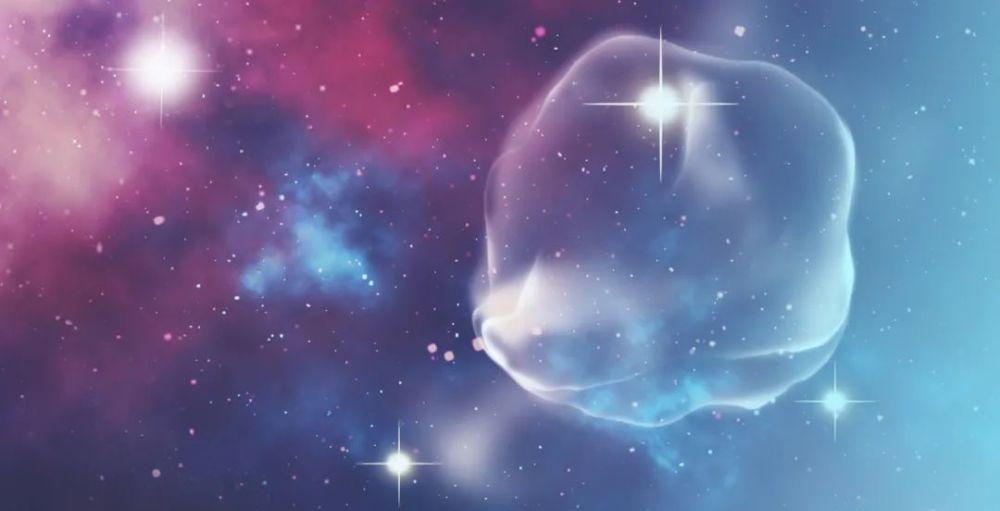
What are the advances in the field of astrophysics in 2021? | Source: pixabay.com
Introduction
Astronomy is undoubtedly one of the oldest disciplines, and astrophysics is the most active part of physics today.
Invited by Intellectuals, Professor Shi Yu of the Department of Physics of Fudan University commented on the progress of various fields of physics in 2021 and published the part of astrophysics and aerospace today. This paper introduces the latest progress in various sub-fields, but also explains the relevant background, such as the Lagrange point and related space missions, and the Exploration of Mars.
Related Reading
Those Advances in Physics in 2021: Particle Physics Section
Written by | Yu Shi (Department of Physics, Fudan University)
Editor-in-charge | Chen Xiaoxue
● ● ●
The review of the progress of this article is roughly divided into 8 parts:
Solar system, telescopes and space stations, electron-shooting volt cosmic ray accelerators, extrasolar planets, neutron stars, black holes, galaxies and cosmology, atomic nuclear reactions within first-generation stars.
01
solar system
Mars exploration
China's first Mars exploration mission was launched in 2013 and approved in January 2016.
On July 23, 2020, the Tianwen-1 probe was successfully launched. The Tianwen-1 probe consists of an orbiter, a lander and a rover (rover).
Tianwen-1 entered Mars orbit in February 2021, landed on Mars in May and dropped the rover Zhu Rong. Zhu Rong traveled more than 1,000 kilometers in four months, and after two months of dormancy (when Mars reached the other side of the sun, communication was interrupted), it toured another 200 meters and obtained a lot of scientific data. The Chinese research team is analyzing climate, geology, and history of the atmosphere in the northern hemisphere of Mars [1].
Located more than a thousand kilometers from the U.S. rover Perseverance, Zhu Rong explores an area called the Utopia Planitia, a basin created by a small object hitting Mars billions of years ago. In 1976, the U.S. Voyager 2 made landfall in the northern part of the basin.
Figure 1 Zhu Rong rover with the landing platform. This picture was taken by the Zhurong Mars rover, corrected and mosaic spliced together to form | source: China National Space Administration, Xinhua News Agency
Zhu Rong is the 6th rover to successfully land on Mars. After two unsuccessful Attempts by the Soviet Union, the United States sent the Rover Sojourner to Mars in 1997. Since then, the United States has sent four more rovers: Spirit, Opportunity, Curiosity, perseverance, the first two of which have stopped working.
Curiosity has been working since 2012. When Perseverance landed on Mars in February 2021, its first mission was to determine whether there had ever been life on Mars. It is also equipped with the Drone Ingenuity. Witty achieved the first powered controlled flight in the extremely thin atmosphere of Mars, becoming the first machine to use power to fly on another planet.
There are other Martian rovers working on Mars, including the U.S. Insight. It landed in November 2018 and began work in February 2019.
Currently, in addition to Tianwen-1, several orbiters are orbiting Mars, including the Odyssey of the United States, which entered orbit in 2001, the Mars Express of Europe launched in 2003, the American Mars Reconnaissance Orbiter (MRO), which entered orbit in 2005, the U.S. Mars Atmosphere and Volatile Evolution Mission (MAVEN), which entered orbit in 2014, and the Mars Orbiter (MOM) of India. The European Space Agency and Russia's Mars Tracking Gas Orbiter (TGO), which entered orbit in 2016, and the UAE's Hope, which entered orbit in 2021.
On December 1, 2021, Zhu Rong conducted a relay communications test with Europe's Mars Express orbiter. On the eve of the Spring Festival in 2022, the Tianwen-1 probe sent back a set of selfie videos.
Star tremors and magnetic fields on Mars
Recently, based on measurements by the U.S. Mars rover InSight, three teams gave results on the internal structure of Mars [2-4]. The results showed that the Flaming Star was active, but the magnitude was lower, mostly below 4, and most of them originated from the star shell. The star's crust is thinner and lacks a mantle mineral layer, so in the early days of Mars, the nucleus cooled faster and soon generated a geomagnetic field. Different regions quickly have temperature differences, driving the fluid to convection, thereby generating an electric current in the initial magnetic field, the current generates a magnetic field, the magnetic field further generates a current, and thus a further magnetic field. The process of generating the magnetic field of Mars has stopped [5]. On Earth, the core of the Earth is cooled by a slower exothermic process that drives fluid convection, resulting in a geomagnetic field. InSight's observations show that the magnetic field generated by Mars in the early days is similar to the current geomagnetic field.
Water on Mars
Mars is cold and dry, and its water is mainly concentrated in polar ice. But its topography shows that it was formed by the impact of water. There are also hydrated minerals on Mars. Therefore, it is speculated that hundreds of millions of years ago, there were rivers and oceans on Mars, and there were conditions suitable for life.
According to previous observations and simulations, it is believed that the water loss on the surface of Mars is mainly through the escape of oxygen atoms and hydrogen atoms (water molecules are composed of these two atoms) to outer space, and the escape of hydrogen atoms is mainly based. Water evaporates into water vapor, and after reaching the middle atmosphere, hydrogen molecules are formed through photochemical processes, transported upwards, and then decomposed into atoms and escaped into outer space. Mars is smaller than Earth, and doesn't have an overall magnetic field shielding the solar wind, so the atmospheric escape rate is higher than Earth's.
The U.S. Mars rover MAVEN and the ESA and Russia's Mars rover TGO measured the current hydrogen escape rate on Mars, as well as the ratio of hydrogen in the remaining water to the content of its isotopes (isotopes refer to nuclei with the same number of protons and different neutron numbers) (deuterium does not escape), thus speculating on the total amount of hydrogen escape in the past. It was found that when Mars is at perihelion, hydrogen escapes the most, and water can be transported directly to the thermosphere and dissociated into atoms.
Dust storms in the lower atmosphere were previously known to occur during the recent period, causing lower convection and facilitating the transport of water to the middle atmosphere. But these results are not enough to explain the loss of water on Mars. Therefore, it has been proposed that there is ice on the subsurface of Mars that has not yet been discovered.
In 2021, it was pointed out that in hydrogen escape, gravitational waves that mainly originate in the lower atmosphere (are fluctuations in the atmosphere, in which gravity plays a role in recovery) lead to fluctuations in temperature and density, strengthen atmospheric circulation, couple the atmosphere of different layers, dust storms allow gravity waves to reach the thermal layer directly, promote water transport upwards, and are crucial in the process of hydrogen escape [6].
But it was also in 2021 that Caltech Scheller et al. proposed that in the first ten to two billion years of Mars, as much as 30 to 99 percent of the water on the surface was chemically weathered into the hydrate in the crust and is now present in hydrated minerals, which they believe may be where most water goes [7-9].
Venus is waterless
There is carbon dioxide in Venus's atmosphere, and its surface temperature can melt lead. Recently, Turbet et al. of the University of Geneva in Switzerland proposed through model calculations that the atmosphere on Venus never condensed into water, but was transported to the dark side to become clouds, absorbing and then emitting the infrared rays emitted by Venus [10-11].
Solar exploration
In 2018, the United States launched the Parker Solar Probe [12-13]. In April 2021, Parker crossed the so-called Alvin plane (a plane where magnetic energy is equal to kinetic energy) into the magnetic-dominated corona, where it began recording data on the sun's magnetic field and plasma. This is an area that has never been directly probed before.
Entering previously unexplored areas means new cognition. When Soviet spacecraft left the Earth's magnetosphere, it was discovered that the solar wind was a stream of supersonic plasma (charged particles).
Pike is still moving closer to the Sun, reaching a 9-fold solar radius in 2025 and detecting a 475-fold brighter sun than in Earth's orbit. A large portion of the incident light is reflected on the surface of the detector, but the surface temperature still reaches 1500 degrees. The detector has a protective cover, the material is carbon, and it has a white porcelain surface. The temperature inside the protective cover can be reduced to 30 degrees. Materials used to detect charged particles in the solar wind are niobium, tungsten, molybdenum and sapphire.
Figure 2 The Pike Solar Probe continues to approach the solar | Source: Johns Hopkins University Applied Physics Laboratory
On October 14, 2021, China launched the Solar Hα Spectroscopy Detection and Dual Superplastic Science and Technology Test Satellite (CHASE) of Nanjing University, known as Xihe [14]. It carries a solar telescope and operates in a sun-synchronous orbit at an altitude of 517 km, studying solar eruptions through the detection and analysis of Hα spectral lines.
Out-of-the-sky rocks
On November 23, 2020, China's Chang'e 5 lunar probe was launched, landing on the moon on December 1 and returning to Earth on December 16. Chang'e 5 brought back 1.7 kilograms of lunar soil, the first lunar soil ever acquired by humans since 1976, from an area previously unexplored by the United States and the Soviet Union. In 2021, Chinese researchers published a series of analyses [15-18] revealing information about the moon's volcanic eruptions and internal thermal history more than 1 billion years ago [19].
There are other news about "extraterrestrial rocks" in 2021 [20].
The United States will publish a recent study of the lunar soil brought back by Apollo 50 years ago (the Apollo program brought back 382 kilograms of rock, rubble, and dirt, but only recently began to study it), and plans to collect lunar soil during the Artemis mission in three years.
The American Perseverance got the rocks of Mars. Japan's deep-space probe Hayabusa2 brought back 5 grams of rock from the asteroid Ryugu. The U.S. OSIRIS-Rex mission took samples from the asteroid Bennu. The United States has had 5 rovers on Mars, but Perseverance collected rock for the first time and brought it back to Earth, and it collected many samples at different locations.
02
Telescopes and space stations
Tiangong space station
In 2021, China began construction of the space station Tiangong in orbit. At present, the Tianhe core module has been launched, and 3 astronauts have been sent to the core module, and the Tianzhou III cargo spacecraft has been launched. After completion, the core module will be docked with a Shenzhou spacecraft, two space experiment modules (Dream Sky and Qingtian) and the cargo spacecraft Tianzhou. More than a thousand experiments will be conducted on the Tiangong space station, including the detection of dark matter, cosmic rays, and gamma-ray bursts [21].
Webb Space Telescope
On December 25, 2021, after years of preparation and the hopes of astronomers around the world, the James Webb space telescope was successfully launched. The project was approved in 1999 and has been in preparation for 10 years [22].
After the launch of the Webb Telescope, it sailed for a month, reaching the halo orbit of the Sun-Earth's second Lagrangian point on January 24, 2022, orbiting this Lagrange point. This Lagrange point is located at an extension of 1.5 million kilometers along the sun-Earth line (150 million km).
Figure 3 On January 24, 2022, the Webb telescope reached the halo orbit of the Sun-Earth's second Lagrange point| Source: Adriana Manrique Gutierrez/NASA
The Webb telescope was folded at launch, and hundreds of engineering operations were carried out after launch, including the unfolding of the instrument and mirror, completing the telescope structure.
The Webb Telescope, man's most complex space observation device to date, is expected to begin observing in July 2022. With a mirror diameter of 6.5 meters and more than 5 times the light collection capacity of Hubble, it can see objects that are a hundred times weaker than the weakest signal that Hubble can see, mainly working in the infrared band, so it focuses on observing the atmospheres of distant objects and exoplanets with a great redshift (redshift value of 20), learning more about the formation of the oldest stars in the universe and the earliest galaxies, and the supermassive black holes at the center of the galaxy.
Review Lagrange Point
For two celestial bodies that orbit each other under the action of Newton's gravitational force, there are 5 equilibrium points, called Lagrange points, on which objects with a mass much smaller than these two celestial bodies can be placed, which are just balanced by the gravitational force of the two celestial bodies and the centrifugal force caused by their own orbital motion. Therefore, artificial satellites are particularly suitable for placement at Lagrange points, requiring very little orbit correction.
Of the five Lagrange points, the fourth (L4) and fifth Lagrange points (L5) form equilateral triangles with these two celestial bodies, respectively, and the balance is stable. The first (L1), second (L2) and third (L3) Lagrange points are on two celestial contiguous lines, and the equilibrium is unstable. The first Lagrange point is between two celestial bodies, close to smaller ones. The second Lagrange point is on the extension of the two celestial lines, close to smaller objects. The third Lagrange point is on the extension of the two celestial bodies, close to the larger objects.
Around each Lagrange point, there is a quasi-periodic (slightly varying per period) Lisaru orbit. Around L1, L2 or L3, there are also periodic halo tracks. In these orbits, objects with much less mass than these two celestial bodies do not require power to operate. These two orbits around L1, L2, or L3 are unstable, but it is easy to achieve orbital position fixation.
For the Sun-Earth (Sun-Earth) system, L1 and L2 are close to Earth. For the Earth-Moon (Earth-Moon) system, L3 is closer to Earth, L1 and L2 are closer to the Moon, and of course the Earth-Moon distance itself is short, so all three Lagrange points can be used. Artificial satellites are often placed in halo orbits or Lisaru orbits at these points, often simply saying that a satellite is at a certain Lagrangian point.
The artificial satellites located in the orbit of L1 Lisaru are:
Advanced Composition Explorer (ACT) for Solar Observation in the United States, launched in 1997;
Genesis, launched in 2001;
3
The U.S. Deep Space Meteorological Observatory (DSCOVR), launched in 2015.
The artificial satellites located in the orbit of L2 Lisaru are:
1 The Wilkinson Microwave Anisotropy Probe (WMAP) of the United States was launched in 2001; the Herschel Space Observatory and Planck Survey of Europe launched in 2009; and the Gaia mission in Europe launched in 2013.
The artificial satellites located in the orbit of the L1 halo wheel are:
The European-American cooperative International Comet Explorer (ISEE-3), launched in 1978, stayed there for several years; and the European-American Cooperative Solar and Heliosphere Explorer (SOHO) launched in December 1995.
The artificial satellites located in the orbit of the Zh-Tsurus L2 halo are:
1 In June 2011, after China's Chang'e-2 completed its lunar exploration mission, it set off from the lunar orbit and entered the halo orbit of L2. Departing in April of the following year, other probes were made, currently as the Sun's first "artificial planet", orbiting the Sun; the U.S. Webb Telescope, launched on January 24, 2022.
The satellites in orbit of the Earth-Moon L1 and L2 Lisaru are:
1 In 2011, the United States will study the Earth's magnetosphere "event time history and sub-storm macroscopic scale action" (THEMIS) satellites through the Earth-Moon L1 and L2 Lisaro orbits to the lunar orbit respectively; on October 24, 2014, China's Chang'e 5 T1 (Lunar Exploration Project III Re-entry Return Flight Tester) was launched, orbiting the moon, the service module and the returner disengaged on November 1, and the returner returned to the ground on the same day. The service module was transferred to the Earth-Moon L2 Lisaro orbit on November 27, making three detours, leaving on January 4, 2015 for a follow-up mission; in 2018, China launched the communication relay satellite Queqiao to the Halo Orbit of Earth-Moon L2. The earth and the far side of the moon can be seen on this satellite at the same time, so in the 1960s, Farquhar, the name of the "halo orbit", suggested that the Apollo lunar landing program set up such a medium-level satellite, which was not implemented. In 2019, Chang'e-4 made a soft landing using Queqiao, sending back images of the far side of the moon.
In addition, on December 17, 2020, the orbiter of Chang'e 5, which completed the lunar exploration mission, separated from the returner, and the returner returned to the ground on the same day, and the orbiter went to the sun-ground L1 to perform the expansion mission. Arrived on March 15, 2021, left in early September (about 1 lap) with no reports of orbits.
Shoot electron volt cosmic ray accelerator
Cosmic rays refer to high-energy charged particles from the Sun or outside the Solar System, with the exception of very few antiparticles, 99% of which are atomic nuclei and 1% of which are electrons. Of these nuclei, 90% are protons (hydrogen nuclei), 9% helium nuclei, and 1% other nuclei. When they pass through the upper atmosphere, collisions with atoms can produce π mesons, etc. The lowest energy cosmic rays come from the sun, the solar wind. High-energy cosmic rays come from outside the solar system. Space telescopes and ground-based observatories have found that the energy of cosmic rays can exceed PeV (beat electron volts), or 1015 eV, or 100 quadrillion electron volts. That's 100 times the energy produced by LHC, the largest accelerator currently available. So where are these "beat electron volt accelerators" located?
The direction of motion of charged particles is altered by interstellar and intergalactic magnetic fields, making it more complicated to understand their origins. It was previously thought that cosmic rays within the Milky Way came mainly from supernova remnants (material thrown by supernova explosions expanding outwards and interacting with the interstellar medium to form particles that derive energy from shock waves). However, it is not clear whether the energy can reach tens of etheric (1 too = 1012) electron volts.
The collision of cosmic rays with matter such as molecular clouds can provide information by producing high-energy gamma rays (i.e., photons) that are 1/10th the energy of the original cosmic rays, but are not affected by magnetic fields because photons are not charged. This helped to find several "Shoot Electron Volt Accelerator" candidates.
In 2016, the High Energy Stereo Telescope System (HESS) collaboration, using telescopes in Namibia, Africa, detected dozens of photons of electron volts from the center of the Milky Way, believing that they were produced by cosmic rays that beat electron volts, the latter related to the supermassive black hole Sgr A* at the center of the Milky Way.
In 2019, the Sino-Japanese cooperative ASγ experimental group at an altitude of 4,300 meters above sea level detected photons of 100 etheric (1012) electron volts from the Crab Nebula. This was later confirmed by the high-altitude Cherenkov (HAWC) Observatory and the cherenkov (MAGIC) telescope for major atmospheric gamma imaging. In 2020, the HAWC Observatory detected the finding of "electron-shooting volts" in several milky ways, one of which is indeed related to supernova remnants.
How are cosmic rays accelerated to shoot electron volts and then produce these gamma rays? HaWC analyzed two examples that showed that cosmic ray protons, after accelerating in a star cluster, collided with the surrounding gas, producing high-energy gamma rays. This challenges the theory based on supernova remnants.
In 2021, the ASγ experiment detected another 0.1 to 1 beat electron volt gamma ray, essentially from the galactic plane of the Milky Way [23,24]. A better explanation for data fitting is that cosmic ray protons collide with interstellar matter, producing π mesons, π meson decay to produce gamma rays.
In 2021, China detected as many as 12 of the most powerful celestial bodies in the Milky Way, the "particle accelerators", with an energy of 1.4PeV [25,26], higher than previously detected. One of them came from the Cygnus direction, consistent with the results of ASγ and HAWC.
These photons are the highest energy photons ever detected by humans.
Figure 4 is located at the high-altitude cosmic ray observatory at Haizi Mountain in Daocheng County, Sichuan Province. The picture is an aerial photograph taken in August 2021| source: ihep.cas.cn/
04
Extrasolar planets
An exoplanet orbiting two stars
The earliest discovered extrasolar planets ( exoplanets ) were discovered in 1992 as planets orbiting neutron stars. Thousands of exoplanets were later discovered, and more planets than stars were found in the Milky Way. Planets influence the motion of their stars, thereby changing the wavelength of light emitted by stars, providing a pathway for indirect detection of exoplanets. The planet of the sun-like star was first discovered using this method, and the discoverer won the 2019 Nobel Prize in Physics. But 1% of exoplanets are discovered through direct imaging.
In December 2021, Janson et al. of Stockholm University in Sweden reported that they had discovered, through direct imaging methods, a massive exoplanet with a mass tens of times the mass of Jupiter, moving around two stars (with a total mass nearly ten times that of the Sun) and reaching a distance of 500 times the distance between the Sun and the Earth, similar to the dwarf planet Sedna at the edge of the solar system, but with a mass a million times that of Sedna [27,28].
This discovery poses a challenge to the theory of planet formation, as the planet is less likely to have formed by the usual process, but may have moved to its current position after forming elsewhere, or formed by gravitational instability (the mass of the protoplanetary disk is too large to cause partial collapse, rather than gathering dust as usual).
Normally, planets form in nuclear accretion. Orbiting new stars, dense gas forms a protoplanetary disk, which then accumulates dust particles and eventually becomes objects tens of kilometers in size, which collide with each other due to gravity and attract nearby debris, eventually forming small planets. If the mass is large enough, it can also attract gases and form an atmosphere.
Satellites in the process of being formed
The star PDS70 has been discovered to have two gas giants (similar to Jupiter). In 2021, Benisty et al. of the University of Grenoble Alps in France used Chile's ALMA (Atacama Large Millimeter and Submillimeter Wave Antenna Array) to discover that one of the planets had a ring of gas and dust, possibly a forming moon [29,30].
05
neutron star
Magnetars, giant flares and fast radio bursts
Extremely short and high-energy instantaneous events of electromagnetic waves, such as gamma-ray bursts, fast radio bursts, and magnetar giant flares, are cutting-edge topics in contemporary astronomy and astrophysics.
Gamma rays, X-rays, and radio waves (also known as radio waves) are all electromagnetic waves, the difference is only the difference in wavelength. Gamma-ray bursts are gamma rays coming from distant galaxies that suddenly increase and weaken for 10 microseconds to a few hours, followed by electromagnetic wave afterglow in other bands, usually thought to come from supernova explosions or neutron star mergers. Fast radio bursts, which are fractions of a microsecond to a few microseconds of radio pulses, were first discovered in 2007 and the cause is unclear. A magnetar giant flare is a burst of gamma rays or X-rays from a magnetar star for about 0.1 seconds.
On April 15, 2020, a short but intense burst of brilliant gamma rays from the neighboring Euphrates galaxy swept across the solar system. A Russian probe aboard the U.S. Odyssey Mars rover first detected the signal. Six minutes later, a solar wind probe between the Sun and earth also recorded the signal. After 5 seconds, the signal reaches detectors near Earth, including the Interplanetary Network (IPN), which consists of several space gamma-ray detectors, the Gamma-ray Burst Surveillance System (GBM) and the Large Area Telescope (LAT) on board the Fermi Gamma-ray Space Telescope. This shining gamma-ray burst is only a few microseconds. In a typical gamma-ray burst, two-thirds of it lasts tens to hundreds of seconds, from massive star explosions in supernova explosions, and one-third shorter than 2 seconds from neutron star collisions. The researchers interpreted this giant flare as a star tremor from a magnetar [33-37].
Magnetars and pulsars are both neutron stars. When a supernova explodes, gravity compresses a star to 20 kilometers, becoming a neutron star, while also compressing the magnetic field to a small range, and the magnetic field strength increases to tens of billions of times, reaching 100 million Tesla. The magnetic field of a magnetar is very strong, thousands of times that of an ordinary neutron star, reaching one hundred billion Teslas. To use an analogy, if a magnetar is located before the Moon and the Earth, our magnetic card will be sucked over. For comparison, the geomagnetic field is 0.00005 Tesla, the magnetic field at sunspots is 0.4 Tesla, and the magnetic field required for magnetic resonance imaging is 10 Tesla.
In 1979, there was a short pulse in the Milky Way that was a hundred times brighter than the usual gamma burst. In 1992, Thompson and Duncan in the United States, and Paczynski in Poland independently interpreted this as magnetar. They proposed that within 10 seconds of the birth of some neutron stars, the fluid inside them churned up, similar to the creation of magnetic fields inside The Earth and Mars, causing the magnetic field to strengthen and become magnetars. They predict that the magnetar's strong magnetic field slows down its self-transformation. This phenomenon was observed by Kouveliotow in 1998. The magnetar's strong magnetic field also causes giant flares. This is used to explain two short, bright gamma bursts in 1998 and 2004.
When the neutron star is formed, after the initial turbulence stage, the outermost few meters of the star shell cools down, in which the heavy atomic nuclei are cooled to form a crystal structure, which has electrons, so it is a benign conductor, which can drive the magnetic field, and the current and magnetic field eventually cause the star shell to flutter, produce cracks and even be destroyed on a large scale, which distorts the external magnetic field of the magnetar, so that the current is increased by a billion times, and even the magnetic field lines can be ejected, resulting in the production of dense gases of positive and negative electrons and photons, and throwing plasma into the surrounding magnetized atmosphere. Plasma emits pulsating X-ray bursts. Under the star shell, the rotation speed of different layers at different depths is not the same, squeezing each other at the interface, under the action of the magnetic field, generating a huge force, causing star tremors, throwing out plasma gas close to the speed of light, containing positive and negative electrons and photons, under the action of the magnetic field, producing short and strong light, that is, giant flares.
Figure 5 Magnetar is a neutron star with a very strong magnetic field| Source: Robert S. Mallozzi, UAH/NASA MSFC
Even in the calm phase, the magnetar is a hundred times brighter than the Sun, and when it erupts, it is trillions of times stronger, and the energy emitted in a fraction of a second can be more energy than usually emitted in 10 years, that is, the energy emitted in a few milliseconds is equivalent to the energy emitted by the sun in 100,000 years. Magnetar flares have been detected several times in the Milky Way, but are too bright to be observed. Recently, such flares have come from outside the Milky Way, allowing astronomers to get a glimpse into the details.
The IPN collaboration (Svinkin et al.) and the GBM co-operative group (Roberts et al.) interpreted the brilliant gamma-ray pulse of April 15, 2020 as a giant flare caused by a starquake near the magnetic pole of a magnetar [33,34]. The IPN co-operative group (Svinkin et al.) also located this event in the neighboring galaxy NGC 253 (the Euphrates galaxy). On this basis, both groups determined the gamma-ray spectrum and temporal details, finding that it was very similar to a known flare, with a rapid process of a few thousandths of a second, and a 10-fold slow decay process. The Fermi-LAT collaboration found that 19 seconds after the gamma rays found in the first two groups, there were several more minutes of higher-energy gamma rays, which were thought to be caused by fast-moving ions [35].
Later, Burns of Louisiana State University identified 3 previously observed short gamma bursts near the Milky Way as magnetar giant flares, bringing the total number of nearby Galaxies to 7. At this ratio, a few percent of short gamma bursts should actually be magnetar giant flares.
Interestingly, 13 days after the giant flare event on April 15, 2020, Canada's radio telescope "Canadian Hydrogen Intensity Imaging Experiment" (CHIME, which detected more than 500 fast radio bursts from 2018 to 2019) and the U.S. "Instantaneous Astronomical Point-Launch Survey 2" telescope observed a fast radio burst, confirming that it was also coming from this magnetar.
Previously observed fast radio bursts were far away, and this time they occurred nearby. This helps clarify the origin of fast radio bursts. Metzger et al. at Columbia University argue that the plasma thrown by the magnetar star tremor causes shock waves that cause electrons to maneuver around the magnetic field, producing rapid radio bursts [70].
Pulsar binary stars accurately test general relativity
A pulsar is a binary star consisting of a pulsar and another object (which can also be a pulsar). As a pulsar moves around the centroid of the binary, it emits gravitational waves, thereby reducing the distance between the two stars and changing the orbital motion of the pulsar, thereby changing the time it takes for the emitted radio pulse to reach the Earth. This provides indirect evidence of the existence of gravitational waves. Hulse and Taylor won the 1993 Nobel Prize in Physics for this discovery.
In December 2021, Kramer et al. of the Max Planck Institute for Radio Astronomy in Germany reported analysis of 2003-2016 data from the pulsar PSR J0737-3039A/B (from six radio telescopes scattered around the world). Discovered in 2003 , the pair are the only known pulsars consisting of two pulsars that are closer to Earth ( 2000 light-years ) and have orbital planes that are well positioned to detect the curvature of space-time [ 31 ,32 ]. Kramer's work improved the test of general relativity to a new level, with an accuracy of one in ten thousand, becoming the most accurate verification of general relativity to date.
Figure 6 Pulsing binary star | Source: M. Kramer/ Max Planck Institute for Radio Astronomy
The energy emitted by the Crab Nebula pulsar
A handful of pulsars occasionally emit giant radio pulses lasting a few microseconds, hundreds to thousands of times brighter than the usual pulses. The pulsars in the Crab Nebula were originally discovered by giant radio pulses. The recent X-ray detector on the International Space Station, the Neutron Inner Composition Explorer (NICER), observed the Crab Nebula pulsar, collected X-ray and radio wave data, and found that X-ray emissions were also increased by 3.8 percent during giant radio pulses, resulting in a total energy tens to hundreds of times greater than previously known .[38]
The heaviest neutron star
NICER measured the mass of the neutron star PSR J0740, which is 2.1 times the mass of the Sun [39,40]. This is the heaviest neutron star known. Nicer directly probes the information to X-rays and then infers the mass. What principle is this based on?
Neutron stars are observed as pulsars with "hot spots" on their surface. As the pulsar rotates, the "hot spot" emits an X-ray beam that rotates like a searchlight. But the gravitational field created by the mass of the neutron star distorts the path of the beam. So from the situation of the X-ray beam changing over time, it is possible to infer the gravitational situation and thus determine the mass.
Multi-messenger studies of neutron stars
Gravity on neutron stars is strong enough to test general relativity. But the equation of state for the matter that makes up neutron stars is unknown. One way to circumvent this barrier is to use universal relations that are independent of the equation of state.
Silva and collaborators of the Max Planck Institute for Gravity in Germany used this universal relationship to obtain the properties of the neutron star's moment of inertia, quadrupolar moment, and surface eccentricity by using the mass and radius given by NICER's X-ray observation data ( as described above ) . The properties of the strong gravitational field were then verified by combining data from the gravitational wave event (GW170817) of neutron stars [41,42].
Thus, the combination of gravitational waves and X-ray observations of neutron stars brings new information about the structure of neutron stars and a new test of general relativity.
06
Black holes
The mass of a supermassive black hole
Previously, the mass of supermassive black holes was determined by indirect methods, such as using the relationship with the large-scale properties of the host galaxy. Supermassive black holes have smaller accretion disks but higher energies, so they are unstable, resulting in randomness in radiation. Burke et al. of the University of Illinois in the United States used the principle that changes in the radiation flux of the accretion disk determine the time scale of attenuation, and found the relationship between the decay time and the mass of the supermassive black hole, thereby determining the mass of the supermassive black hole [43,44].
Supermassive black holes make neutrinos
Only a dozen neutrinos from the depths of the universe are detected each year. IceCube, a neutrino detector located at the South Pole, uses 1 cubic kilometer of Antarctic ice to assemble a photon detector to calculate the direction of neutrinos according to the arrival time and brightness, and determine whether they come from nearby or deep in the universe. In 2017, IceCube traced a neutrino to a blazar, a superluminous galaxy in which a supermassive black hole sucks into objects and spews out beams of particles, including neutrinos . This neutrino was previously the only neutrino from the depths of the universe (not the solar neutrino) that had previously been identified as a source.
On October 1, 2019, IceCube detected another neutrino candidate, possibly from the depths of the universe, sending a message to observing astronomers. California's Zwicky Transient Facility determined that the neutrino came from a known tidal disruption event (TDE): a supermassive black hole 7.5 billion light-years away tore a star apart [46,45].
The discovery of these two cosmic neutrinos suggests that the jets of supermassive black holes are a major source of high-energy (up to the pat electron volts) neutrinos deep in the universe. High-energy neutrinos in the universe are produced from very energetic protons, so TDE is also a source of high-energy cosmic rays.
Galactic jets and magnetic fields
Almost all galaxies have supermassive black holes at their centers, millions to billions of times more massive than the Sun. The centers of galaxies near us are not active, but some galaxies are very active, sucking in material and emitting various electromagnetic waves with huge amounts of energy. Some galaxies thus emit two very strong jets, producing electromagnetic waves, known as radio galaxies. Theoretically, the generation, convergence and shape of jets are determined by magnetic fields. Specifically, electrons near supermassive black holes approach the speed of light, swirling in the magnetic field, emitting electromagnetic waves. Particles converging by magnetic fields form jets that can be millions or even tens of millions of light-years long, up to a hundred times the size of the Milky Way. But the previous evidence is limited.
In 2021, Chibueze et al. used the MeerKAT Radio Telescope in South Africa ( one of the most sensitive radio telescopes ) to obtain high-resolution images of the radio galaxy MRC0600-399 , found the diffusion region of the radio emission near the nearly 90-degree bending point of the jet stream , and confirmed with computer simulations that supersonic jets do occur in the bending layer of the magnetic field [47,48]. This result suggests that in a chaotic galaxy cluster environment, there is an ordered strong magnetic field. This helps to understand the magnetic field and gas dynamics during galaxy clumping.
Black holes in dwarf galaxies
LIGO and Virgo announced the gravitational wave signal GW190521 last year, interpreting it as two black holes with masses of 65 and 85 solar masses merging to form a black hole with a mass of 142 solar masses .[49] But theoretically, a black hole formed by a supernova explosion cannot have a mass between 65 and 135 solar masses. If the mass is greater than 65 solar masses, then the light inside the star produces positive and negative electron pairs, thus losing the light pressure that supports the outer layer of the star, the outer layer collapses, accelerates the nuclear reaction, and the star disappears, so it cannot form a black hole. If the mass is greater than 135 solar masses, the star collapses directly into a black hole. 85 solar masses happen to be in this forbidden zone. The combined black hole with a mass that is neither larger than a black hole from a star nor as massive as a supermassive black hole (hundreds of thousands to billions of solar masses), is the first black hole to be found in this intermediate mass range.
In 2021, Palmese et al. of Fermilab proposed that the two black holes were at the center of two dwarf galaxies (low-mass galaxies) to avoid rewriting the theory of black hole formation [50,51]. This image can be used to study the early formation of supermassive black holes and the evolution of galaxies.
The limits of information emitted by black holes
In 1981, Jacob Bekenstein deduced from the law of causation and the second law of thermodynamics that there was an upper limit to the rate at which information was emitted by physical systems. Shahar Hod deforms it to a limit on relaxation time and illustrates the process that can be used for black hole relaxation to equilibrium.
In April 2021, Carullo et al. of the University of Pisa verified that the conjugated black holes observed by the Gravitational-Wave Observatory met this limit. Of all the observed black hole merger gravitational wave events, they selected eight that could accurately determine the relaxation time, and for the black holes generated by the merger, calculated the information emissivity per unit of energy, reaching an upper limit of 75%, the fastest known physical system [52,53].
07
Galaxies and cosmology
Galaxy spiral arm
70% of galaxies are spiral galaxies, including the Milky Way. According to lin jiaqiao and Xu Jinghua's density wave theory, the vortex structure can only appear in stable rotating disks, not in young galaxies. Previously, spiral arms were found in galaxies 11.3 billion years ago, about 2.5 billion years after the Big Bang.
In 2021, Tsukui and Iguchi of the National Astronomical Observatory of Japan proposed that a galaxy had a spiral arm 1.4 billion years after the Big Bang, based on data from the LAMA Radio Telescope in Chile [62,63].
Cosmic information brought by pulsar timing arrays
The time for RF pulses from millisecond pulsars to reach Earth fluctuates. If this fluctuation is caused by a change in the distance of space caused by gravitational waves, then the time fluctuations of different pulsars are related. The purpose of the pulsar timing array is to monitor these fluctuations and thus detect gravitational waves of very low frequencies (1-100 nanohertz). Theoretically, these gravitational waves could come from supermassive black holes, cosmic strings, early cosmic phase transitions, and even quantum fluctuations in very early gravitational fields amplified by cosmic inflation.
In December 2020, the NanoGrav collaboration in North America released observations of 45 pulsars over a 12.5-year period. At the time, some people interpreted the signal as gravitational waves, speculating that they originated from cosmic strings or primordial black holes.
More recently, the NANOGrav collaboration itself attributed this to gravitational waves caused by phase transitions at low energies (10MeV) in the early universe. This phase transition is based on particle physics theory that goes beyond the Standard Model [54,55]. A similar signal was detected by the PPTA cooperative group in Australia, but thought it was noise [56].
BICEP/Keck and inflation models
Modern cosmology holds that as the universe expanded, the electromagnetic waves that flooded the early universe became the background radiation of today's cosmic microwaves, while the large-scale structure of the universe came from early density fluctuations. These density fluctuations cause primordial gravitational waves.
In the 1980s, the inflationary model proposed to solve the standard cosmological difficulties suggested that the universe underwent a sharp exponential expansion in the very short time after the Big Bang. The cosmic inflation causes primordial gravitational waves to produce B-modes in the cosmic microwave background radiation. B-mode is a behavior of electric field polarization, meaning that the relationship between electric fields in different directions is similar to the behavior of a magnetic field (commonly used as B). However, dust in the universe can also cause this consequence.
The purpose of the BICEP (Polarized Background Imaging of Pangaxies Outside the Milky Way) project at the South Pole is to measure the polarization of the cosmic microwave background radiation and look for B-mode. In 2014, BICEP published B-mode results, which were thought to be caused by primordial gravitational waves, but later clarified that dust came from the Milky Way.
Now THE 3 INSTRUMENTS OF BICEP, TOGETHER WITH THE NEARBY KECK ARRAY, WORK TOGETHER TO FORM BICEP/KECK. Recently, they have given limits on the contribution of gravitational waves by excluding the dust factor. The key quantity is the so-called tensor-scalar ratio r, which represents the amplitude ratio of gravitational waves to density waves. Recently they announced that r was less than 0.036, refreshing the previous Planck satellites' 0.11 and BICEP's 0.09 and 0.07 [57,58].
There are many versions of the inflation model. The results of BICEP/Keck exclude certain versions. However, most versions of the inflationary model predict that r is greater than one in ten thousand. BICEP/Keck and several other similar experiments are expected to achieve the precision needed to measure smaller r-values, and Japan will launch a satellite, LiteBIRD, for this purpose in 2028. B-mode results at high precision, whether negative or positive, can cause major changes in cosmological theory.
Early dark energy
In previous years, data from supernovae suggested that the universe was expanding 5-10% faster than previously believed. Therefore, it has been proposed that "early dark energy" existed for the first 300,000 years after the Big Bang.
The Atacama Cosmological Telescope (ACT) collaboration and another group analyzed data from the Act in Chile, respectively, for 2013-2016 and concluded that signs of "early dark energy" were found. If correct, the age of the universe would be changed from 13.8 billion years to 12.4 billion years [59-61].
But this is only a preliminary result, pending further testing by the ACT and the Antarctic Telescope. The two telescopes are used to measure the fluctuations of the cosmic microwave background radiation (CMB). Previously, the most accurate data for the CMB came from the Planck satellite, which ESA worked from 2009 to 2013.
Anti-star
If there are anti-matter anti-stars in the universe, then there will be matter-antimatter annihilation into gamma rays.
Dupourque et al. at the University of Toulouse in France screened 5787 gamma-ray sources and proposed an upper limit: up to 2.5 antistars per million stars [68,69].
08
Nuclear reactions within the first generation of stars
Ancient stars, the so-called metal-poor stars (which contain very little element other than hydrogen and helium), have particularly high levels of calcium, which is theoretically attributable to weak supernova explosions that occurred after the death of the first generation of stars (the so-called third-generation stars), and the calcium in the first generation of stars originated from a series of nuclear processes called prison escape reactions, starting with the capture of protons by the fluorine nucleus, which produced photons and neon. Through this reaction, the star "breaks" from the carbon-nitrogen-oxygen cycle, resulting in the production of calcium. The carbon-nitrogen-oxygen cycle refers to a cycle of nuclear reactions in which carbon, oxygen, and nitrogen act as catalysts, and the net result is that 4 protons are converted into 1 α particle (i.e., helium nucleus), 2 positrons, 2 electron neutrinos, and photons.
The ability to escape depends not only on the abundance of fluorine, but also on the ratio of this reaction of fluorine to protons to another reaction (producing α particles and oxygen, including 3 channels: only these two particles; accompanying the production of photons; and concomitant production of π mesons). This ratio is greater than 8, and the theory of calcium production and weak supernovae can be established.
Fluorine is a peculiar element in the universe, and it is easy to react nuclearly with the rich proton and helium nuclei (that is, α particles) in the universe, except for the prison escape reaction in the first generation of stars, which is absent from the main atomic nuclear reactions in stars.
In 2021, de Boer et al. at the University of Rotterdam in the United States analyzed 70 years of data on the two nuclear reactions of fluorine and protons and found that there was a large uncertainty in the reaction rate of the prison break reaction (producing photons and neon), which created uncertainty about the weak supernova model and the origin of calcium [64,65].
Also in 2021, the Jinping Deep Earth Nuclear Astrophysical Experiment (JUNA), led by the China Academy of Atomic Energy, accurately measured the reaction rate of α particles and oxygen (accompanied by photon production) [66,67].
Expect them to work hard to measure the rate of the jailbreak reaction (which produces photons and neon) and the reaction rate of the other two channels that produce α particles and oxygen (only these two particles; concomitantly produce π mesons).
bibliography:
[1] Smriti Mallapaty, CHINA’S MARS ROVER HAS AMASSED REAMS OF GEOLOGICAL DATA, Nature | Vol 600 | 9 December 2021 | 203
[2] B. Knapmeyer-Endrun et al., Science 373, 438 (2021).
[3] A. Khan et al., Science 373, 434 (2021).
[4] S.C. St hler et al., Science 373, 443 (2021).
[5] Sanne Cottaar and Paula Koelemeijer, The interior of Mars revealed, Science 373 (2021) 388.
[6] Hiroyuki Kurokawa, Hydrated crust stores Mars’ missing water,Science 372, 27 (2021).
[7] Research highlights, Nature 591, 504 (2021).
[8] Erdal Yi it, Martian water escape and internal waves, Science 374, 1323 (2021) .
[9] E. L. SCHELLER et al., Long-term drying of Mars by sequestration of ocean-scale volumes of water in the crust, SCIENCE 372, 56-62 (2021)
[10] Turbet, M. et al. Nature 598, 276–280 (2021).
[11] James F. Kasting, Chester E. Harman, Nature 598 | 14 October 2021 | 259
[12] How to Survive Flying Too Close to the Sun, Michael Schirber, December 14, 2021 | Physics 14, 176
[13] Christina M. S. Cohen, Momentous Crossing of a Solar Boundary, December 14, 2021 | Physics 14, 177
[14] Here comes the Xihe! The first solar exploration science and technology test satellite of the mainland was successfully launched! Nanjing University News, 2021-10-18.
[15] Li, Q.-L. et al. Nature 600, 54–58 (2021).
[16] Che, X. et al. Science 374, 887–890 (2021).
[17] Tian, H.-C. et al. Nature 600, 59–63 (2021).
[18] Hu, S. et al. Nature 600, 49–53 (2021).
[19] Richard W. Carlson, Robotic sample return reveals lunar secrets, Nature 600, 2 December 2021, 39
[20] Katherine Wright, Rockin’ Time for Space Missions, April 12, 2021 | Physics 14, 55A
[21] Smriti Mallapaty, CHINA’S SPACE STATIONIS PREPARING TO HOST 1,000 SCIENTIFIC EXPERIMENTS Nature 596, 20 (2021).
[22] Daniel Clery, First light machine, Science 374, 807 (2021).
[23] M. Amenomori et al. (Tibet ASg Collaboration), “First detection of sub-PeV diffuse gamma rays from the Galactic disk: Evidence for ubiquitous galactic cosmic rays beyond PeV energies,” Phys. Rev. Lett. 126, 141101 (2021).
[24] Petra Huentemeyer, Signs of PeVatrons in Gamma-Ray Haze, April 5, 2021, Physics 14, 41.
[25] LHAASO Collaboration. Nature 594, 33–36 (2021).
[26] Petra Huentemeyer, Hunting the strongest accelerators in our Galaxy, Nature 594, 30 (2021).
[27] Janson, M. et al. Nature 600, 231–234 (2021).
[28] Kaitlin Kratter, Giant planet imaged orbiting two massive stars, Nature 600, ( 2021) 227.
[29] M. Benisty et al., Astrophys. J. 916, L2 (2021).
[30] Research Highlight, Nature 596, (2021) 11.
[33] Roberts, O. J. et al. Nature 589, 207–210 (2021).
[34] Svinkin, D. et al, Nature 589, 211–213 (2021).
[35] The Fermi-LAT Collaboration, Nature Astron. 5, 385–391 (2021). [36] Christopher Thompson,Nature 589, 199–200 (2021).
[37] Joshua Sokol, A STAR IS TORN, Science 372, 120 (2021).
[70] Daniel Clery, Galactic flash points to source of enigmatic fast radio bursts, Science 368, 1171 (2020).
[31] M. Kramer et al., “Strong-field gravity tests with the double pulsar,” Phys. Rev. X 11, 041050 (2021).
[32] Lijing Shao, General Relativity Withstands Double Pulsar’s Scrutiny, Physics 14, 173, December 13, 2021
[38] Teruaki Enoto et al., Enhanced x-ray emission coinciding with giant radio pulses from the Crab Pulsar, Science 372, 187–190 (2021) .
[39] Thomas E. Riley et al, A NICER View of the Massive Pulsar PSR J0740+6620 Informed by Radio Timing and XMM-Newton Spectroscopy, 2021, The Astrophysical Journal Letters 918 L27
[40] Matteo Rini, Sizing Up the Most Massive Neutron Star, April 29, 2021 | Physics 14, 64.
[41] H. O. Silva et al., “Astrophysical and theoretical physics implications from multimessenger neutron star observations,” Phys. Rev. Lett. 126, 181101 (2021).
[42] Daniela Doneva, May 3, 2021 | Physics 14, 66
[43] C. J. Burke et al., Science 373, 789 (2021).
[44] Paulina Lira and Patricia Arevalo, How massive is that black hole? SCIENCE13 AUGUST 2021 VOL 373 ISSUE 6556 735
[45] Daniel Clery, Rare cosmic neutrino traced to star-shredding black hole,
SCIENCE 872 26 FEBRUARY 2021 VOL 371 ISSUE 6532.
[46] Robert Stein et al., A tidal disruption event coincident with a high-energy neutrino, Nature Astronomy volume 5, pages510–518 (2021).
[47] Chibueze, J. O. et al., Black hole jets bent by magnetic fields, Nature 593, 47–50 (2021).
[48] Joydeep Bagchi, Black hole jets bent by magnetic fields, Nature 593, 40 (2021)
[49] Rosalba Perna, A Heavyweight Merger, September 2, 2020 Physics 13, 111
[50] A. Palmese and C. J. Conselice, “GW190521 from the merger of ultradwarf galaxies,” Phys. Rev. Lett. 126, 181103 (2021).
[51] Rachel Berkowitz, Do Merging Dwarf Galaxies Explain a Peculiar Gravitational-Wave Detection? May 5, 2021 | Physics 14, s52
[62] TAKAFUMI TSUKUI, SATORU IGUCHI, Spiral morphology in an intensely star-forming disk galaxy more than 12 billion years ago, SCIENCE 372 (2021)1201-1205
[63] Michael Schirber, Spiral Arms in an Infant Galaxy, May 28, 2021 | Physics 14, 81
[52] G. Carullo et al., “Bekenstein-Hod universal bound on information emission rate is obeyed by LIGO-Virgo binary black hole remnants,” Phys. Rev. Lett. 126, 161102 (2021).
[53] Christopher Crockett, Black Holes Obey Information-Emission Limits, April 22, 2021 | Physics 14, s47
[54] Z. Arzoumanian et al. (NANOGrav Collaboration), “Searching for gravitational waves from cosmological phase transitions with the NANOGrav 12.5-year dataset,” Phys. Rev. Lett. 127, 251302 (2021).
[55] Michael Schirber, Pulsars Probe Early Universe, December 15, 2021, Physics 14, s160.
[56] X. Xue et al. (The PPTA Collaboration), “Constraining cosmological phase transitions with the Parkes pulsar timing array,” Phys. Rev. Lett. 127, 251303 (2021).
[57] P. A. R. Ade et al. (BICEP/Keck Collaboration), “Improved constraints on primordial gravitational waves using Planck, WMAP, and BICEP/Keck observations through the 2018 observing season,” Phys. Rev. Lett. 127, 151301 (2021).
[58] Daniel Meerburg, Squeezing down the Theory Space for Cosmic Inflation, October 4, 2021 | Physics 14, 135
[59] Hill, J. C. et al., The Atacama Cosmology Telescope: Constraints on Pre-Recombination Early Dark Energy, ))https://arxiv.org/abs/2109.04451
[60] Poulin, V., Smith, T.L. & Bartlett, A., Dark energy at early times and ACT data: A larger Hubble constant without late-time priors, Phys. Rev. D 104, 123550
(2021).
[61] Davide Castelvecchi,NEW DARK ENERGY COULD SOLVE UNIVERSE EXPANSION MYSTERY, Nature 597, 460 (2021).
[68] .S. Dupourqué et al., “Constraints on the antistar fraction in the
Solar System neighborhood from the 10-year Fermi Large Area Telescope gamma-ray source catalog,” Phys. Rev. D 103, 083016 (2021).
[69] Rachel Berkowitz, Counting All the Antistars in the Sky April 20, 2021 | Physics 14, s50
[64] R. deBoer et al., “19F(p, g)20Ne and 19F(p, ag)16O reaction rates and their effect on calcium production in Population III stars from hot CNO breakout,” Phys. Rev. C 103, 055815 (2021)
[65] Michael Schirber, Uncertainty over First Stars, May 26, 2021 | Physics 14, s66
[66] L. Y. Zhang et al., “Direct measurement of the astrophysical 19F(p, ag)16O reaction in the deepest operational underground laboratory,” Phys. Rev. Lett. 127, 152702 (2021).
[67] Christopher Crockett, Pinning Down the Fate of Fluorine The first results from the Jinping Underground Nuclear Astrophysics particle accelerator refine a key reaction rate for the destruction of fluorine in stars. October 7, 2021 | Physics 14, s124
Plate editor| Ginger Duck