Today we finally entered the most critical content, what is the internal structure of the hadron? You see, in the previous article, I have introduced eight baryons with a spin of 1/2, including protons, neutrons, Σ+, Σ0, Σ-, Λ, Ξ0, Ξ-.
There are also nine baryons with a spin of 3/2, including: Δ++, Δ+, Δ0, Δ-, as well as Σ*-, Σ*0, and Σ*+, as well as Ξ*- and Ξ*0.
These are baryons, and there are 7 mesons with a spin of 0, including: π+, π0, π-, and K0, K+, K-, and anti-K0, which are 7.
There are also 9 mesons with a spin of 1, including ρ+, ρ0, ρ-, as well as K*0, K*+, K*-, anti-K*0, and ω and Φ. That's all the particles we introduced earlier, they're all hadrons.
They are all involved in strong interactions, so many particles, so scientists began to wonder, these particles have no internal structure, always feel that they can not be elementary particles, because these particles are not only numerous, but also very chaotic, only from the symmetry, and aesthetic point of view, these particles must be composed of several elementary particles.
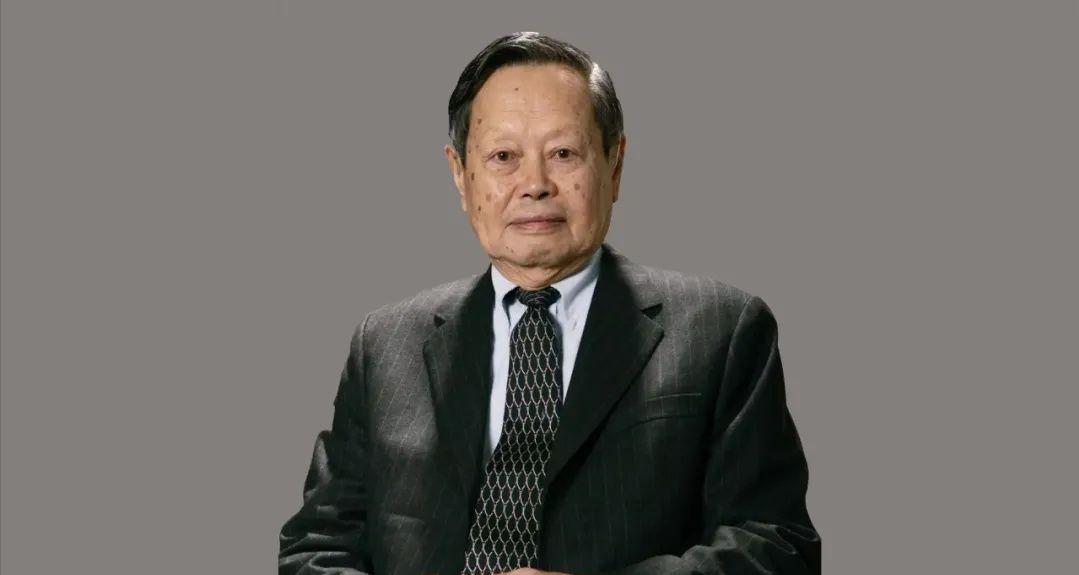
In fact, the earliest time to think about the internal structure of hadrons can be traced back to the 40s, when we only know that there are five kinds of hadrons: proton neutrons, π +, π0 and π-, when Mr. Yang Zhenning was a student of Fermi at the University of Chicago, they co-wrote a paper proposing the Fermi-Young model, arguing that protons and neutrons are elementary particles, and the other three π mesons are composed of protons and neutrons, such as π+ is composed of a proton and an anti-neutron, and π- is composed of a neutron and an antiproton;
Although this model is wrong, but at the time this model seems to be no problem, you see, you can use the quantum numbers we said earlier to test it, such as the baryon number of protons is 1, the number of anti-neutrons is -1, the combined baryon number is 0, the number of baryons of the π + meson is 0, and the charge, isospine these quantum numbers are all consistent.
It wasn't until we discovered Σ+, Σ0, Σ-, Λ and Ξ0, Ξ-, as well as K0, K+, K-, anti-K0, these hadrons, that we found that the Fermi-Young model was incorrect, because these new particles have a new quantum number called singular number, and protons and neutrons do not have singular numbers, so they cannot be composed of these singular particles.
By 1955, Japan's Sakata Shoichi had decided that since a singular number was needed, it was enough to treat one of the singular particles as an elementary particle, so Sakata said that protons, neutrons, and Λ were elementary particles, and that they constituted all known hadrons.
Sakata's model can not only explain K0, K+, K- and inverse K0, as well as π+, π0, π-, the quantum numbers that these mesons have, such as K+ are composed of protons and inverse Λ, in which the charge, baryon number, isospin, singular number, these quantum numbers are not problematic.
And in Sakata's model, according to the combination of protons, neutrons and Λ, new mesons were also predicted: η, it turned out that this new particle really existed, which means that we now have eight kinds of mesons with a spin of 0, and since the spin is 0, we also call them pseudo-standard mesons.
Unfortunately, Sakata's model cannot explain Σ+, Σ0, Σ- and Ξ0, Ξ- quantum numbers of several baryons, which shows that Sakata's model is also wrong.
It can be seen that whether it is the Fermi-Young model or the Sakata model, it is intended to use the quantum numbers of several elementary particles to form the quantum numbers of other particles.
Therefore, the physicist Gell-Mann felt that we should not consider what this elementary particle is, but first classify the quantum numbers of the existing hadrons to see what the relationship between them is.
According to the above ideas, Gell-Mann drew a Y-I₃ with I₃ as the abscissa and Y as the ordinate, after reading the previous article, you should know that this Y is the superchart, equal to S+B, I₃ is a very important quantum number in the strong interaction, it is the value of the projection on the third component of the isospin.
Okay, so let's put the eight kinds of pseudo-standard mesons into the Y-I₃ diagram, which is what we see now, this is the pseudo-standard meson octet state, then we put the vector meson into the Y-I₃ diagram, and that's it.
This is called the vector meson octet state, and we have 8 baryon protons, neutrons, Σ+, Σ0, Σ-, Λ and Ξ0, Ξ-, and the same way to put them into the Y-I₃ graph, it is like this.
This is called baryon octafold state, it can be seen that these hadrons have reflected a good symmetry, before we said that some of these baryons are isospine multimorphic states, such as protons and neutrons are isospine duostates, there are two kinds of charges, Σ+, Σ0 and Σ- are the triplet state of the isocentric spin, there are three charge states, Ξ0, Ξ- is the isotropic spin double state, there are two kinds of charges, Λ is the isotopic spin singlet state.
But now when we mark them on the Y-I₃ diagram, we find that these eight baryons have greater symmetry, that is, baryon octets.
In addition, we have 9 kinds of baryons, and after putting them on the Y-I₃ diagram, this is the case.
It can be seen that if the bottom particle is missing in this picture, it will appear asymmetrical, and if you add that particle, it will not become a baryon decaunder state.
The question is, does the particle at the bottom exist, and if it exists, what are the quantum numbers? This is very simple, from the above figure we can find some laws, from top to bottom each row of Y number will be decremented by one, which indicates that the position of the particle Y = -2, at the same time we can calculate its S = -3, we can also see Δ++, Σ +, Ξ0, the charge of this hypotenuse is decreasing, so we speculate that the charge number of this unknown particle Q= -1, at the same time we can also calculate its spin is 3/2, we call this new particle Ω-.
Miraculously, the periodic table once arranged the chemical elements clearly, but also predicted new elements, and now Gell-I₃ arranged the hadrons clearly with the Y-I₃ diagram, and can also predict new particles.
In order to find the Ω faster - we need to know what its mass is, we can know how much energy it takes to produce it, then in the baryon octet state, and the decatal state, the mass of these particles also has a certain relationship, then Gell-Mann calculated the mass of Ω based on the mass of other baryons is about 1683 Mev.
In 1964, the Brookhaven Laboratory in the United States, through the collision of K- and protons, found the figure of Ω- in the bubble chamber, the measured mass of Ω- was 1672 Mev, the lifespan was 0.821×10^-10 seconds, it can be seen that the decay of this particle is controlled by a weak force, and the discovery of Ω- also verified Gell-Mann's theory, and he also won the Nobel Prize in 1969.
But that's not the end of it, because we haven't unveiled the internal structure of hadrons yet, but Gell-Mann's classification of hadrons has made the messy particles regular, and we only need to theoretically assume a few new particles that can explain the quantum numbers of all hadrons.
Then Gell-Mann modified sakata's model to still be three elementary particles, but they are no longer protons, neutrons, and Λ particles, because the quantum numbers of these three particles cannot constitute the quantum numbers of other baryons.
So Gell-Mann hypothesized that there are three elementary particles, upper quark, lower quark, and odd quark, denoted as u, d, and s, and their baryon numbers are 1/3, spins are 1/2, the charge is 2/3, -1/3, -1/3, and the singular number is 0, 0, and 1.
These three elementary particles also have antiparticles, anti-upper quarks, anti-lower quarks, and anti-odd quarks, and as long as these six quarks have some of the above quantum numbers, they can make up all the hadrons known so far. That's why I've been emphasizing quantum numbers, because the quark model is all about quantum numbers.
The mediator is composed of a quark and an antiquark, of which the baryons are composed of three quarks, which is the quark model proposed by Gell-Mann and Zweig in 1964.
When this model was proposed, many people did not accept it at that time, because it had a strange fractional charge, that according to previous experience, the charge carried by the elementary particle of the electron was the basic unit of the charge, but now Gell-Mann proposed a fractional charge, so it felt very strange.
So for a long time people have been looking for quarks, and in the end they have found nothing, but there are still a few evidences that quarks are real, such as in SLAC in 1968, experimenters bombarded protons with high-energy electrons, and found that electrons have a large angle of deflection, which indicates that the electron hits something small in the proton, this experiment is similar to Rutherford's experiment on α particles, which can only indicate that quarks may exist, but this does not exactly mean that this is quark.
And we didn't find any examples of fractional charges in later high-energy scattering experiments, as well as in oil drop experiments in Miregent, so people wonder why we can't find free quarks if quarks really exist. We answer this question when we talk about force. The next lesson, we'll find out about other quarks.