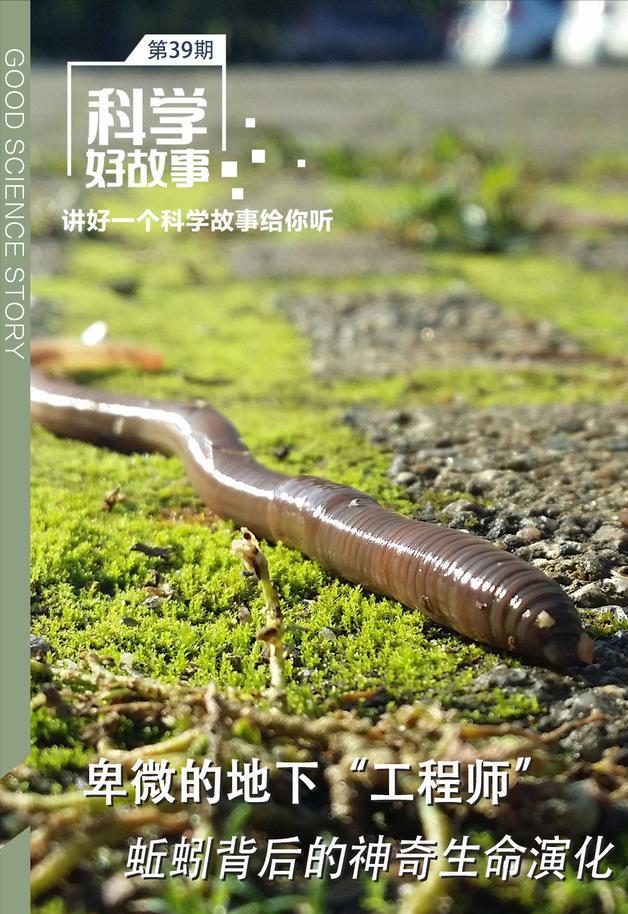
Source: Aeon
Author: Kevin Laland
Translator: Ren Tian
Living things do not evolve blindly under forces beyond their control, but at the same time evolve, they also shape and influence the environment itself.
Human activities are profoundly influencing the evolution of life on Earth. Not only have we led to the ongoing mass extinction, but we have also forced animals, plants, and fungi to constantly adapt to our man-made world. For example, today's urban birds need to sing in a higher pitch to be able to drown out the noise of traffic.
On the other hand, while cultural knowledge and engineering techniques have improved humans' ability to accelerate environmental change, this trend is common to all species. When you put some bacteria in a Petri dish, they will produce nutrient-rich byproducts that new bacterial strains can take advantage of, rapidly proliferating and evolving large new microbial populations. Ponds built by beavers become breeding grounds for ducks; webs abandoned by spiders are used as hibernation sites by ground insects; and plants can improve the soil through substances secreted by their roots. The ways in which organisms change their environments in turn alters the evolutionary pressures they and other organisms face during survival and reproduction. In other words, their behavior affects the outcome of natural selection. This process, known as "niche construction," is present in all species, though their effects tend to be milder and more limited than those of humans.
However, in the field of evolutionary biology, niche construction theories are controversial, in part because the mechanisms of natural selection have traditionally been considered "blind": natural selection has continually shaped organisms over thousands of years, adapting them to certain niches without deviating from their goals or wills. Humans go through the same shaping process, but it is generally accepted that we are active actors who shape the environment we adapt to, rather than evolving to adapt to existing niches.
Breeding ground for the northern Gannet (Morus bassanus) on the German island of Helgoland
Our brains evolved so well to process linguistically encoded information and learn knowledge because we established and adapted to a rich cultural domain; we domesticated plants and animals, and by incorporating them into our diets, triggered the selection of genes that metabolize these foods; we invented agriculture, promoted population growth, and inadvertently made choices about genetic resistance to population diseases such as typhoid or cholera. Our parents not only passed on their genes to us, but also left behind a world that had already been changed by them. This heredity in the ecological sense means that humans evolved not in response to a static adaptive environment, but by shaping the environment, reducing or reinforcing the specific selection pressure it gives us. As evolutionary biologists Richard Levins and Richard Levantine put it: "Organisms are both objects of natural selection and creators of conditions of natural selection, influencing their own evolution." ”
A static, bio-independent, adaptive terrain in which time moves downwards. Image adapted from Tanaka et al (2020)
Fundamentally, the fundamental point of this debate is that scholars disagree on the extent to which other organisms can also guide evolution by modifying adaptive environments. Terrain metaphors may help to understand the process of evolution. You can imagine an adaptation topographic map that shows the relationship between an organism's fitness (ability to survive and reproduce) and one or more traits of the organism.
For example, in a bird population, one axis of the fitness terrain represents body size and the other axis represents tail length (or we can substitute traits for the frequency of related genes). The adaptability terrain is presented on a three-dimensional surface, and well-adapted birds have the best combination of body size and tail length, and are at the "peak" of adaptation; birds with less adaptability are in "troughs". The highest areas in the adaptable terrain represent creatures with the best body size and tail, which have the strongest survivability. A bird population has a range of different traits, each combined to be a different point in the topographic map. Through natural selection, the population gradually converges to the combination of traits that are most suitable for the local habitat, and in the adaptation topographic map, this process is presented as the population climbing up along the peak of local adaptation. There's more to climb to the top of fitness, though: you can change your traits to help you reach the top, or you can move the peak closer to you.
Humble niche builders
Beneath our feet are some humble but very powerful niche builders. Earthworms are "ecosystem engineers", a species that alters the physical and chemical environment and in doing so affects ecosystem function. Curiously, despite their ecological success, they are not actually suitable for living on land from an anatomic point of view. They have been on land for tens of millions, if not hundreds of millions of years, but still retain the basic physiological functions of the ancestors of freshwater worms. Unlike most terrestrial animals, earthworms produce a lot of diluted urine — similar to freshwater organisms — which makes them easy to die of dryness: if earthworms are dug out of the soil in the sun, they will soon be sunburned. So, how did these animals survive on land for so long with such poor structural adaptability?
The answer is niche construction. Earthworms did not evolve physiological characteristics adapted to the terrestrial environment, but instead created an aquatic world on land. Their success stems directly from their own "gardening skills". Earthworms are excavated in a way that reduces the "matricidal potential" of the soil, i.e. the soil's ability to retain water. This makes it easier for earthworms to absorb water and replenish the moisture lost due to excretion. In other words, earthworms efficiently build a "paddling pool" that keeps itself damp.
In this way, and with a variety of other "tricks," earthworms tilt the adaptive terrain in their favor, with a striking effect that is strangely reminiscent of scenes of human invasion of the original natural environment. European earthworms (i.e., common earthworm Lumbricus terrestris) are now considered the main drivers of forest plant community changes in North America. In the common impression, earthworms are good animals for the soil, but they can also become invasive species. In North America, European earthworms are having a devastating impact on hardwood forests. The earthworm fauna native to North America was almost completely extinct during the Pleistocene glacial period, and many ecosystems were devoid of earthworms until the arrival of European colonists in the 16th and 17th centuries. With the introduction of the European earthworm, the species spread rapidly, and while they invaded deciduous forests, they also caused significant changes in soil properties, putting native species of flora and fauna at risk.
Earthworms drag organic matter into the soil as food or material to fill holes, which causes the litter layer to be rapidly lost, resulting in a warmer, drier soil surface. Their activities also alter the rate at which chemical elements such as carbon and nitrogen circulate in ecosystems, such as accelerating the decomposition of plant matter; their excavations also increase soil ventilation and drainage. These changes usually occur in the 10 years after the invasion, opening up another state that lasts for decades. Due to the historical lack of earthworms, the surface litter layers of these forests contain large amounts of carbon. Just as humans use fossil fuels, when earthworms remove this litter, large amounts of stored carbon are released into the atmosphere in the form of carbon dioxide, exacerbating global warming.
This change in habitat quality has profound implications for other species. Studies in recent years have found that plant diversity declines as the abundance of introduced earthworm ecological taxa increases, with native plants being hit the hardest and soil invertebrates decreasing with increased earthworm biomass. The local flora and fauna have adapted to an environment free of earthworms, which is why they suffer. At the same time, there are a number of species that benefit: like human interference, earthworm "engineering" promotes the invasion and reproduction of fast-growing bacteria, grasses and sedges, as well as some large animals such as deer. Earthworm burrows are used as a resting place by invertebrates and even some vertebrates (such as salamanders), and their dung mounds (vermicules) are hotspots for microbial activity. Earthworms also usually coexist with invasive shrubs such as rat plums and honeysuckle. Part of the reason is that earthworms change the soil's "seed bank" — naturally stored seeds — by eating the seeds and choosing the size of the seeds. The seeds that survive being eaten are concentrated in earthworm manure, benefiting from the nutritional and physical protection of this natural fertilizer. In general, it is non-native plants that dominate.
It is therefore not surprising that the ecological chain reaction triggered by the invasion of earthworms has repercussions in forest ecosystems. Some researchers even worry that earthworms could trigger an "invasion collapse," in which non-native species accelerate their invasion of each other. This may not be so intuitive: earthworms themselves benefit from their "engineering" and the resulting invasions of other species, which allows them to build new fitness peaks on topographic maps of fitness. Earthworms promote the growth of invasive shrubs, which in turn promote the growth of earthworm populations. Because shrubs can produce a higher quality defoliation layer (lower carbon-nitrogen ratio), which produces a self-perpetuating feedback loop, this is also favored by the European earthworm. It can be said that earthworms are effectively engaged in agriculture, from preparing the soil to adjusting the seed bank to using the earthworm mound to promote the germination of their favorite crops. They have cultivated similar reciprocal relationships with deer, pigs and microbes. Interestingly, the diversity of earthworm species is correlated with earthworm biomass, suggesting that the "engineering" of European earthworms is also beneficial to other earthworm species.
Earthworms also build homes to accumulate chances of evolution in their favor. There are more than 6,000 species of earthworms in the world, and their ecology and behavior vary greatly. However, most earthworm species are not just "nomads" who wander around and forage in the soil, leaving the used land behind. Instead, earthworms build semi-permanent caves, and these "buildings" usually exist longer than their lifetime. Charles Darwin once pointed out that earthworm caves are not simply excavated, but fairly strong vertical tunnels, sometimes even more than a meter deep, and "lined with cement". This "cement" is compacted from the secretions and excrement of earthworms and forms a unique, one-centimeter-thick cave lining called a "drilosphere." Every earthworm cave – their living area – has a pile of manure on the surface of the soil at the opening, the vermiculite mound, which is both a kitchen and a toilet, consisting of litter and feces. Earthworms even dig small side holes for cocooning. Many earthworms come out at night in search of food and mates, but do not leave the cave, but often leave the end of their tail in the cave and quickly retreat to safety as soon as the situation arises.
Digging a hole is an energy-consuming activity – it's much easier to occupy and renovate a former occupant's house than it is to build it yourself. It is generally believed that earthworms do not dig holes longer than the width of their bodies, but smaller earthworms are often found in larger caves, suggesting that either juveniles inherit their parents' caves or that scattered individuals make their homes in empty caves. Long-term field observations have shown that the same cave has been used for a long time far longer than the life cycle of earthworms. Therefore, it is not difficult to see that earthworms seem to prefer soils that have previously been inhabited by their kind rather than "undeveloped" soils.
Improved soil, readily available shelter and a more adequate food supply are not all that earthworms leave for future generations. Each cocoon of an earthworm inherits a microbiome from the gut of its parents. In fact, the environment of earthworms includes not only the soil and surrounding organisms, but also the bacteria, fungi and single-celled eukaryotes that live in the digestive system of earthworms and the surrounding earthworm contact circles. Again, this arrangement is reciprocal: earthworms provide a food-rich and mobile environment for soil microbes, giving them the opportunity to ferment in an oxygen-deficient environment and spread through the soil, and the presence of these symbiotes also improves the adaptability of earthworms.
Niche construction and evolution
Like humans, earthworms have established their own niche. The environment in which they live is not fixed, but remains dynamic and changing according to the activities of their inhabitants. Not only have earthworms changed the soil, they were also growing crops, releasing greenhouse gases, building houses, congregating into cities and spreading to the countryside, just like humans.
Earthworms aren't the only species that improve their environment through niche construction: Seaweed secretes sticky chemicals to bond sand to keep the environment stable; Bush bushes and pine trees in North America spark forest fires by spreading flammable conifers, pine cones and pine oil to burn competitors; and some ants spray acidic chemicals or plant fungal crops to wipe out trees that are not suitable for their habitat.
Given the way organisms improve their environment according to their needs, do we need to rethink the adaptive terrain model? From the most radical perspective of niche construction, the answer is yes: organisms are not just adapting, but collectively guiding evolution. For example, beavers have a much more extensive impact than the pools they build. They are both firefighters and irrigation managers, dramatically improving the upstream ecosystem to withstand climate disturbances such as fires and droughts. In this way, beavers change the local environment, and thus the way natural selection works on itself and other species. They are part of a network of feedback and causal loops in which previously selected organisms drive environmental changes, and biologically altered environments subsequently select changes in the organism.
Biologically suppressed adaptability terrain; maps over time from top to bottom. Image adapted from Tanaka et al (2020)
Niche construction overlaps with several other concepts in ecology and biological evolution, notably "ecosystem engineering" and "dynamics of ecological evolution.". But the niche-building theory is unique in that it claims that changes in the environment by organisms (niche construction) and the legacy they acquire over time (ecological inheritance) are evolutionary processes– both of which lead to evolutionary changes.
What exactly is the evolutionary process? The jury is still out, although most textbooks focus on phenomena that directly alter the frequency of genes — such as natural selection, mutation, or random genetic drift (random changes in the composition of population gene banks). Going back to the metaphor of adaptive terrain, these phenomena are all forces that drive biological populations between peaks and valleys. In contrast, niche construction theory advocates a broader concept of evolutionary causation.
Conventional wisdom holders might argue that while niche construction "can influence or even lead to evolutionary processes of natural selection," it is "not an evolutionary process in itself, at best like an ever-changing environment" (says evolutionary biologist Douglas Futuyma). This view ignores the difference between niche construction and general environmental change, that is, niche construction changes natural selection in an orderly, directional and continuous manner. It not only changes, but also guides adaptive evolution by imposing a certain statistically significant tendency on the direction and intensity of choice. Organisms create landscapes that adapt to the environment. For example, in a study on the effects of earthworm invasion on native plant communities in 15 forests in the northeastern United States, the results showed surprisingly consistent patterns. Despite differences in earthworm species and abundance, native plant composition and characteristics, and geographic region, the same response associated with earthworms was detected at all sites.
Perhaps, as Richard Levantine suggests, rather than imagining evolution as an adaptive terrain with fixed peaks and valleys, imagine the process of organisms walking on a trampoline. But the example of earthworms also shows that during evolution, organisms not only inhibited their own adaptability, but also improved their environment, which in turn improved their adaptability relative to their later environment. This "engineering" is enough for us to make judgments about the outcome of evolution. Earthworms, for example, did not reach the adaptive peak of the physiological functions of typical terrestrial animals; instead, they undertook another evolutionary journey, reaching an unlikely peak of adaptation that exhibited aquatic physiological functions on land.
Recently, a team of theoretical biologists and philosophers designed a new mathematical framework to simulate natural selection and niche construction. They managed to describe the evolution of two connected adaptive terrains (rather than a single one): biological evolution corresponded to climbing to the top of the mountain in the adaptive terrain, while environmental evolution corresponded to climbing to the top of the mountain in the "constructed terrain". The relationship between the two determines how successful a particular organism will ultimately be. Just as we cannot fully understand the evolution of secondary sexual characteristics such as male peacock tails without tracking the co-evolution of female peacock mating preferences; without niche construction, it is impossible to describe the evolution of adaptive traits with biological changes in environmental characteristics. Using their niche-building evolutionary framework, the researchers analyzed the dynamics of the evolution of three microbes, all of which are involved in different forms of niche construction.
Mathematical analysis of niche construction
The description of how natural selection drives increased population fitness is commonly referred to as Fisher's Fundamental Theorem for Natural Selection: an increase in population fitness caused by natural selection equals an additive genetic variance in fitness (i.e., the number of genetic variants that increase differences in survival and reproduction). British statistician and geneticist Ronald Fisher argues that since the fitness of populations cannot increase indefinitely, this process must be balanced by corresponding environmental degradation. Coupled terrain models mathematically show that this conservation of fitness does not necessarily hold under niche construction. Organisms can improve their absolute fitness by changing their environment (such as building mounds on a mountain), or they can reduce their adaptability by destroying the environment (such as digging pits).
This model can help us understand the evolution of earthworms and other species that reshape the environment, including humans. The great success of European earthworms in North American forests is probably not a manifestation of natural selection adapting earthworms to the forest environment, but the result of earthworms by changing the forest ecology to make them more suitable for themselves. The same applies to humans. The domestication of plants, the cultivation of soil, the introduction of new irrigation methods, the application of fertilizers, etc., have greatly enhanced the ability of the land to feed human beings. Through repeated environmental improvements, our own absolute fitness has been greatly improved – just as we build higher and higher mountains on the adaptable terrain and climb them.
Evolutionary ecologists Tobias Uller and Haike Highlander devised a way to incorporate niche construction into another universal evolutionary model, the "Price equation," named after legendary population geneticist George Price. The equation is based on two core evolutionary processes: natural selection of traits over time, and biased transmission (or inheritance) of traits across multiple generations. These processes can all be defined and measured with statistics, which in turn allows for calculating changes in traits (i.e., evolution) over time, and how much each role selection and transfer play in them. The selection terms in the equation are given by the product of the trait's heritability (the extent to which genetic differences contribute to the variability of traits) and selection differences (a measure of the extent to which traits affect fitness). For example, if a large dog has more offspring than a puppy, but a dog with upright ears has a similar number of offspring to a dog with droopy ears, the choice of size varies greatly, while the choice of ear shape differs less. Traits can be anything, including fitness. Since heritability is related to the number of genetic variations, when the trait we focus on is fitness, the selection terms in Price's equation are roughly equivalent to Fisher's fundamental theorem of natural selection.
In contrast, the genetic terms in the equation represent factors other than direct selection that affect how traits change over time, including the bias of transmission. Interestingly, Price refers to the term as an "environmental change item" that seems to apply specifically to earthworms. Earthworms do not alter the soil arbitrarily, nor do they ruthlessly degrade the soil. On the contrary, generation after generation of earthworms have not only improved soil quality for themselves, improved their adaptability through niche construction, but also left a biased ecological legacy for future generations. Just as Fisher hypothesized that the environment would deteriorate, researchers in the past have tended to assume that the transitive bias term in Price's equation may be negative when the trait of interest is fitness. This is both because mutations are often harmful to organisms and because environmental influences are often (mistakenly) not hereditary. Thus, relatively speaking, the role of processes related to transmission in evolution is overlooked. When organisms construct or choose a better environment for their offspring, they may inadvertently lead to adaptive evolution – even in the absence of natural selection.
Tobias Uller and Haiki Hirandra used the Price equation to further point out how niche construction affects evolution. In evolutionary analysis, heritability — the extent to which traits differ due to genetic differences — is often estimated by comparing statistics on trait similarity between parents and offspring, such as parent-child regression, such as whether large dogs produce larger puppies. The transmission (inheritance) of biologically improved environments between generations affects the similarity of offspring to their parents, which tends to overestimate estimates of heritability.
Price's equation simulates evolutionary changes caused by natural selection (which can be broken down into differences in heritability and selection) and non-selective factors such as transfer bias, i.e., offspring can inherit an environment that has been improved by their parents. Niche construction can influence these three components of evolution, and earthworms are a prime example
Earthworms again provide a good example. Earthworms have a slimy appearance because they secrete mucus to protect the outer skin, and changes in mucus secretion are sensitive to soil properties. Measuring the amount of mucus secreted by earthworms under different soil conditions reveals a strong correlation in mucus levels between parents and offspring. However, this is not because earthworms pass on genes that "produce more mucus" to their offspring. This intergenerational similarity and high heritability is mainly inherited through ecological inheritance rather than genetic inheritance: offspring "inherit" the soil (and microbiome) of their parents and therefore produce similar amounts of mucus. If the researchers investigated how the mucus levels of invading earthworms responded to natural selection, they might find significant trait changes — meaning a choice of lower mucus secretion. But in reality, this may be the ecological effect of earthworms in ecological niche construction for future generations to improve the soil environment. Earthworms do not exhibit a genetic response to natural selection, but traits have undergone systematic and directional changes in a way that is closely related to fitness.
Another way that niche construction influences evolution is by modifying the effect of traits on fitness (e.g., altering selection differences, which is part of the first choice in Price's equation). We can use the earthworm's tendency to migrate as a trait that can change when encountering harsh soil conditions or inadequate food supplies. Experimental studies have shown that earthworms can reduce their own (and other earthworm species) migration needs by improving habitat quality. Instead of migrating to more fertile pastures, they have completely transformed their original habitats through irrigation, fertilization and recultivation.
Now, let's consider the (hypothetical) adaptation of "fast" and "slow" dispersers in invasive earthworm populations. Fast-dispersers may multiply in the soil, but the initial fitness is low because the original soil quality is poor. However, as the environment improves, they attract more earthworms, as well as (beneficial to them) invade shrubs and animals, further improving their health. Eventually, earthworm populations became so dense that their adaptability declined due to competition and overexploitation of the soil, triggering a new wave of dispersal. In contrast, slow-scatterers had a higher degree of relative fitness than settlers in the beginning.
The "seesaw" type of adaptive terrain, where organisms change the direction of adaptation; the maps change over time from top to bottom. Image adapted from Tanaka et al (2020)
However, as the soil quality of the invaded forest improves, the relative suitability of the slow disperser decreases until they are too dispersed. Driven by niche construction and competition, fast and slow spreaders are locked in a cyclical battle. Returning to the metaphor of adaptive terrain, the terrain at this time is like a seesaw: slow dispersion is advantageous, but as the fast disperser improves the soil so that the seesaw tilts in another direction, rapid dispersion gradually becomes a peak of adaptability until the competition flips the seesaw back again.
All of these mathematical analyses help us understand the results of the way organisms change their environment. To grasp adaptive evolution, it is necessary not only to understand how niche construction evolves by natural selection, but also to understand that the environmental roots of natural selection themselves change through niche construction. We need to extend models of adaptive terrain to truly understand the positive and immediate environmental changes made by organisms. Evolving populations are less like mindless climbers, desperately climbing the peaks of fitness and more like industrious landscape architects, equipped with excavation and construction tools that can reshape the adaptive terrain for their own purposes. Today, as human niche construction and ecological heritage continue to destroy the planet's ecology, we may learn from humble animals such as earthworms to learn how to make the world we live in a better place. (Ren Tian)