<h1 class="pgc-h-arrow-right" > introduction</h1>
Surface plasmon excitons are collective electromagnetic oscillation patterns formed by the coupling of free charge and electromagnetic waves, capable of manipulating the interaction of light and matter at the nanoscale. The dispersion patterns of plasmons in different metal structures depend on their spatial dimensions and have been studied in depth in fundamental physics and applied techniques. Sheng Wang of the University of California, Berkeley, and his mentor Feng Wang and collaborators recently reported hybrid plasma excitons from the heterostructure of mixed-dimensional van der Waals formations from one-dimensional carbon nanotubes and two-dimensional graphene formations.[1] Metal carbon nanotubes have quantum properties that are distinct from conventional plasma excitons, and their plasma exciton properties are independent of carrier density, so they cannot be regulated by gate voltage.[2][3][4] In contrast, the plasma excitator wavelength in the carbon nanotube/graphene heterostructure can be continuously regulated by the gate voltage with a regulation amplitude of up to 75%, while maintaining the excellent characteristics of the one-dimensional system of medium ex vitro exciter superspace compression and low loss. This shows that the heterostructure of the mixed-dimensional van der Waals can realize electrically regulatable plasma excitator nanowires with various functions. The results were published in the international journal Nature Communications.[1]
First authors: Sheng Wang, Seok Jae Yoo, Sihan Zhao, Berkeley
Corresponding authors: Wang Sheng, Seok Jae Yoo, Wang Feng, Berkeley
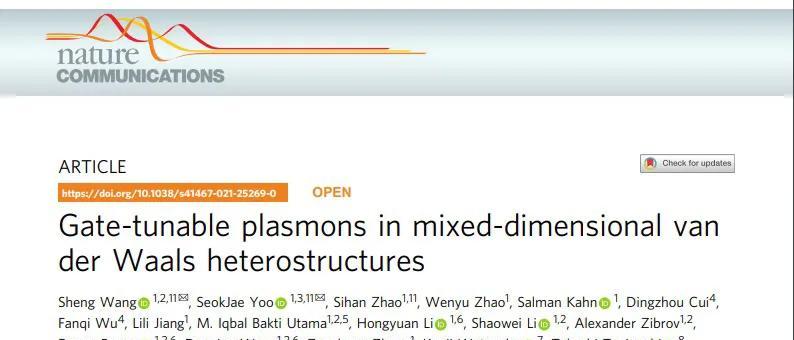
< h1 class="pgc-h-arrow-right" > research background</h1>
Surface plasmon excitons are collective oscillation modes formed by the coupling of free charge and electromagnetic waves, and can regulate the interaction between light and matter at the nanoscale beyond the diffraction limit. The spatial dimensions of material systems have a profound impact on the properties of plasma excitons. In one-dimensional materials such as carbon nanotubes, strongly correlated interactions between electrons form Luttinger liquids, resulting in one-dimensional Luttinger liquid systems exhibiting special quantum plasma exciton properties. In metallic carbon nanotubes, plasma excitons combine excellent characteristics such as non-dispersive propagation speed, deep sub-wavelength locality, and low loss, but cannot be regulated by the gate voltage because the quantum plasma excitons in this system do not change with carrier concentrations.[2][3][4] The plasma excitons in two-dimensional graphene encapsulated in a two-dimensional film of boron nitride can be well regulated by the gate voltage. The coupling of plasma excitons between materials of different dimensions can greatly change the dispersive properties of plasma excitons and present new properties, but the pattern of plasma excitons in such mixed-dimensional materials has not yet been detected.
< H1 class="pgc-h-arrow-right" > innovative research</h1>
In view of this, Sheng Wang of the University of California, Berkeley, his mentor Feng Wang and collaborators designed and prepared the van der Waal heterostructure of the carbon nanotube/boron nitride/graphene hybrid dimension and studied the strong coupling of carbon nanotubes plasma excitons and graphene plasma excitons in the hybrid heterostructure. Highlights are as follows:
Highlight 1. Drawing on the dry transfer stacking technology based on temperature-controlled viscous plastic film in two-dimensional materials, the clean carbon nanotube/boron nitride/graphene hybrid heterostructure was successfully prepared controllably, and the hybrid plasma excitator mode in the system was systematically studied with the highly sensitive scanning near-field light microscope built by himself.
Figure 1: Infrared nanoscale imaging of carbon nanotubes (SWNT)/boron nitride (h-BN)/graphene (Graphene) hybrid heterogeneous structures with ex vivo excitons.
a schematic of infrared nano-imaging of medium ex vivo excitons of SWNT/h-BN/Graphene heterostructures. The hybrid-dimensional heterostructure of the design is achieved by dry transfer stacking technology based on temperature-controlled viscous plastic films, with a top-down layout of SWNT/Top h-BN/Graphene/Bottom h-BN/SiO2/Si. Graphene carrier density can be continuously regulated by the applied gate voltage Vg. To achieve infrared nano-imaging based on scanning near-field light microscopy, an infrared laser with a wavelength of 10.6 μm is used to irradiate the tip tip of the atomic force microscope and collect elastic scattered light from the tip. b The boundaries of the graphene and the top h-BN layer are outlined with black and green dotted lines, respectively. The overlapping region between graphene and the top h-BN, as well as the carbon nanotubes at its top (optically invisible), constitute the SWNT/h-BN/Graphene heterostructure. c , d Height image of representative areas of heterostructure and corresponding near-field images. M1 and M2 in c are metallic carbon nanotubes with bright contrast in near-field images due to excitation of plasma excitons, while S in c are semiconductor carbon nanotubes with negligible near-field response due to the lack of free electrons.
Highlight 2. The wavelength of the graphene plasma exciton is electrically regulated by the gate voltage to match the wavelength of the metal carbon nanotube and the neutral excitator, thereby achieving a strong coupling of the two plasma exciton modes. The hybrid plasma exciton formed by the strong coupling combines the characteristics of carbon nanotube plasma excitator deep sub-wavelength locality and low loss, and also has the characteristics of graphene plasma excitator electrically adjustable. These characteristics are difficult to possess by a single system of intermediate ex vivo excitons, so this mixed-dimensional plasma exciton system can achieve electrically adjustable nano-optics with various excellent properties.
Figure 2: Gate voltage-controlled plasma excitons in carbon nanotube (SWNT)/boron nitride (h-BN)/graphene (Graphene) heterostructures.
a Long SWNT M1 height image. b – i The corresponding near-field image of SWNT M1 at different gate voltages from 40 to -100 V. The double stripes parallel to the carbon nanotubes arise from interference between the excitation of the tip of the needle and the graphene plasma excitation wave reflected by the carbon nanotube. As the gate voltage increases, the double stripes become more pronounced and more separated. This evolution shows that the graphene carrier density and the corresponding graphene plasma exciton can be continuously adjusted by the applied gate voltage. We clearly observed significant near-field signal oscillations near the ends of carbon nanotubes, and they are sensitively dependent on gate voltages. The plasma excitator wavelength λp is equal to twice the oscillation period in the near-field image, is marked by a white double arrow (d and i), and becomes longer as the gate voltage moves away from 0V. j Short SWNT M2 height image. K – s SWNT M2 corresponds to a near-field image at various gate voltages from 40 to -120 V. SWNT M2 acts as a Fabry-Perot plasma excitator nanocave, in which the propagated plasma excitons reflect back and forth at both ends of the nanocavity and produce a collective response. From m-s, the number of wave-annumabs is reduced from 7 to 4, and the realization of the plasma wave exciton regulation amplitude is about 75%.
bibliography
[1] Wang, Sheng et al. Gate-tunable plasmons in mixed-dimensional van der Waals heterostructures. Nature Communications 12, 5039 (2021). https://doi.org/10.1038/s41467-021-25269-0
[2] Wang, Sheng, et al. "Nonlinear Luttinger liquid plasmons in semiconducting single-walled carbon nanotubes." Nature Materials 19, 986-991 (2020).
https://doi.org/10.1038/s41563-020-0652-5
And the previous article of this public account "Nonlinear Ratinger Liquid Plasma Excitators in Semiconductor Carbon Nanotubes".
[3] Wang, Sheng, et al. "Logarithm Diameter Scaling and Carrier Density Independence of One-Dimensional Luttinger Liquid Plasmon." Nano Letters 4, 2360-2365 (2019):2360-2365.
https://pubs.acs.org/doi/10.1021/acs.nanolett.8b05031
[4] Wang, Sheng, et al. "Metallic Carbon Nanotube Nanocavities as Ultra-compact and Low-loss Fabry-Perot Plasmonic Resonators." Nano Letters 4, 2695-2702 (2020).
https://pubs.acs.org/doi/abs/10.1021/acs.nanolett.0c00315
and the previous article of this public account "NanoLett.: Metal Carbon Nanotube Nanoclumen: Ultra-Compact and Low Loss Fabry-Perot Plasma Oscillator".
Article links
https://doi.org/10.1038/s41467-021-25269-0
This article is transferred from:
https://mp.weixin.qq.com/s/4e2wo8OnMiGt6WADQ_4AXw
For more cutting-edge news, welcome to search for the public account:
Isometric radical frontier