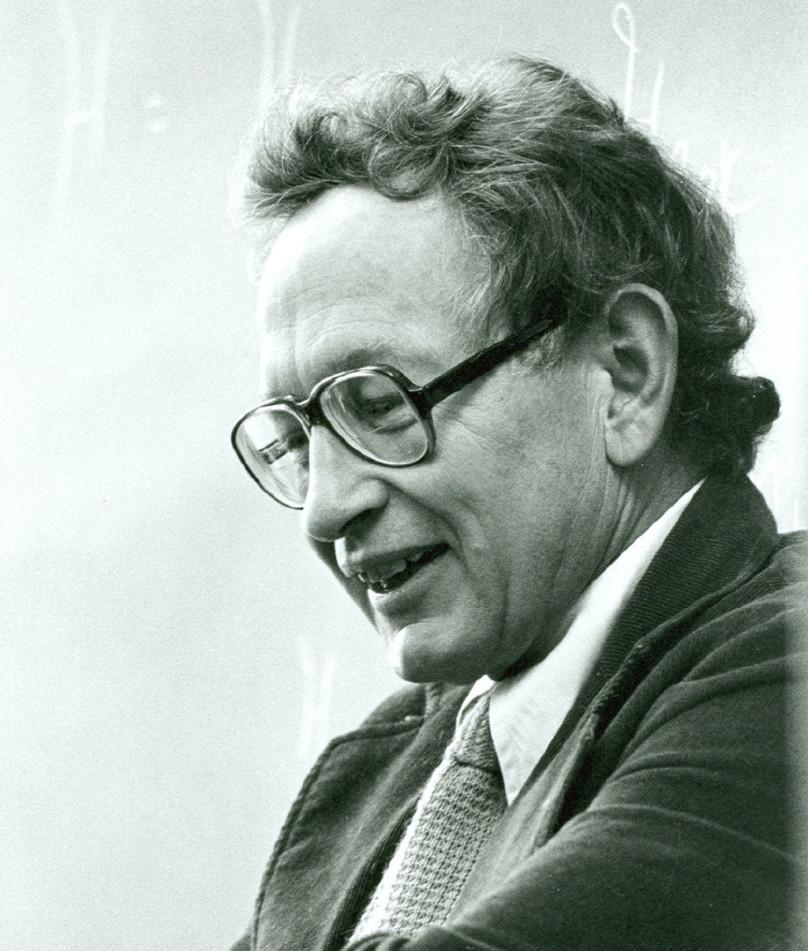
Physicist P.W. Anderson won the 1977 Nobel Prize in Physics for his work on disordered and magnetic materials, but that wasn't enough to highlight his contribution. He promoted the fusion of multibody theory and solid state physics, and eventually formed today's condensed matter physics, which can be called the grandmaster of condensed matter physics. In the classic paper "More is Different," Anderson refutes the long-pervasive reductionist view of physics, arguing that new complexities emerge from nature at different scales. After that, he participated in the creation of the Santa Fe Institute, a holy place for complex systems research. Anderson died on March 29, 2020, with profound effects on condensed matter and beyond making him one of the brightest stars in the sky of theoretical physics.
Written by | Andrew Zangwill
Translate | Dong Weiyuan
Review | Liang Jin
Philip Warren Anderson (1923-2020) was one of the most accomplished and important physicists of the 20th century. In more than 50 years of research at Bell Labs, Cambridge University and Princeton University, he has been striving to explore the laws of nature with great taste, profound insight and amazing creativity.
Anderson promoted the fusion of many-body theory with solid state physics, culminating in the formation of today's condensed matter physics, and his contributions to this area far exceeded those of others. In his 1984 book, Basic Notions of Condensed Matter Physics, he argued that a better way to describe the behavior of a system containing 1023 particles would be to build and use the Hamiltonian quantities of the model than to decipher the multibody Schrödinger equation. Over the past few decades, this method has become mainstream in textbooks of condensed matter physics of all kinds.
Another Nobel laureate, Pierre-Gilles de Gennes, was so envious of Anderson that he once called him "the pope of solid state physics." The nickname is quite appropriate, because Anderson does seem to have established the doctrinal creeds of the field. His loyal followers are constantly watching his voice, and many will try to speculate on his point of view and try to prove it. Yet in his own view, he was a rebel, never obedient, always filled with an insatiable curiosity about the reasons behind the laws of nature. In this article, I will briefly introduce Anderson's life and scientific work, hoping to make readers feel the many influences he has brought to us.
1. Son of the Earth
Anderson's parents, whose ancestors were Scottish and Irish immigrants, fought in the American Revolutionary War and later ran farms on fertile land west indiana. Farm work was clearly not everyone's favorite, and Anderson's grandfather and uncle preferred to teach Latin, math and English at Wabash College in Crawfordsville. The same hobby led Anderson's father and uncle to become plant pathologists. Anderson's father was a professor at the University of Illinois at Urbana-Champaign, where he also grew up. During this time, he would return to Crawfordsville frequently and maintain close ties with his family. His family has a typical Indiana temperament, both questionable and meticulous and sensitive.
Figure 1. 10-year-old Anderson (front row center) with family and relatives (1934). | Source: Susan Anderson
In high school, Anderson excelled in his studies but also excelled in running, tennis, and speed skating. He also participated in biology and chess clubs, participated in school performances every year, and created and recited works at graduation ceremonies to show his good historical accomplishment. His high school graduation photo album reads "The Importance of Being Earnest," the name of a play by writer Oscar Wilde, Chinese translated as "No Play."
During those years, Anderson often followed his father to weekend parties in college. Faculty members at the University of Illinois often get together on weekends to hike, swim, play baseball, and they often talk about politics with left-wing ideas. These ideas deeply influenced the young Anderson, leading him to a lifelong passion for social justice and justice. It was through these meetups that Anderson became acquainted with F. Anderson, the head of the Department of Physics. Wheeler Loomis, and won a scholarship to Harvard University at his recommendation.
In the second year of Anderson University, the United States entered World War II. Eager to contribute to his country, he moved from the physics department to the electronic physics major that could graduate quickly, a special major set by Harvard to encourage students to join the military. After graduation, he spent two years as a microwave engineer at the U.S. Naval Research Center laboratory, an experience that made him realize that he should develop his skills in theoretical physics. After the war, Anderson returned to his alma mater to pursue a Doctorate, and he realized that Harvard still owed him an orthodox education in physics, and that quantum theory was never mentioned in the electronic physics course.
Many wartime students focus only on military-related knowledge and skills, while peacetime requirements for knowledge and skills vary. As a result, many students with theoretical research qualities came to Harvard like Anderson to recreate. Eleven of them chose to follow the university's newly hired star professor, Julian Schwinger, to work on atomic physics. At this time, Anderson showed his frequent anti-mainstream spirit since then, deliberately found an excuse that he did not like atomic physics, turned to John Van Vleck, and completed the complete quantum mechanical theoretical calculation of the microwave absorption spectrum of small molecules for the first time.
Anderson's doctoral dissertation, completed in 1949, is still widely cited today, but his job search process has been difficult. Interviewers don't take much interest in a candidate who is familiar with molecular physics, and they seem to prefer experts in atomic physics. In the end, Anderson accepted a position with the help of his teacher and joined the research group led by William Shockley of Bell Labs to work on solid state physics, which was the only research group in the United States at that time focused on this field.
2. Bell Labs
For 50 years in the mid-20th century, Bell Labs was probably the best research institution in the world. Anderson benefited from the environment there, and the lab benefited greatly from his addition. During his first few months, he immersed himself in the 1940 book The Modern Theory of Solids by Frederick Seitz, confirming Shockley's conjecture about the origin of ferroelectricity in barium titanate oxide ceramics, and recommending to an academic journal club Linus Pauling's paper explaining metal bindings in terms of resonant valence bond theory.
Yet, like many of his predecessors, Anderson was soon disillusioned with Shockley's brutal style and turned to three other distinguished scholars at Bell Labs, Gregory Wannier, Conyers Herring, and Charles Kittel. Wannier taught him the love of statistical mechanics, Herring taught him not only solid state physics, but also many general encyclopedic knowledge of literature, Kittel taught him magnetism and specifically recommended that he study antiferromagnetism (Kittel is the classic textbook of solid state physics, Introduction to Solid State Physics). There has been a lack of direct experimental means in this regard, and the magnetic neutron scattering technology that has just emerged at that time can be used for antiferromagnetic experimental research.
Figure 2. Anderson's mentors at Bell Labs Gregory Wannier, Conyers Herring and Charles Kittel. | Image credit: Wannier portrait courtesy of the AIP Emilio Segrè Visual Archives, Physics Today Collection; Herring and Kittel portraits courtesy of the AIP Emilio Segrè Visual
In January 1952, Anderson submitted to the Physical Review a paper on estimating antiferromagnetic quantum theory, which was of considerable historical significance, and it was in this article that the concept of spontaneous symmetry breaking was first proposed. This is a very peculiar phenomenon, the system's Hamiltonian representation clearly has some symmetry, but the system will spontaneously choose one of several simplified symmetrical configurations and thus lose symmetry. Anderson discussed this a lot, including something similar to what later became known as the Goldstone mode. Unfortunately, it was not until 10 years later that his vision of symmetry breaking did not get attention.
After meeting Japanese theoretical physicist Ryogo Kubo, Anderson was quickly invited. Kubo invited him to Tokyo for the first International Conference on Theoretical Physics held there and visited Kubo's research group for 6 months. Bell Labs granted Anderson unpaid leave, and in September 1953 he arrived in Japan with his wife, Joyce, and daughter Susan.
Figure 3. In January 1954, Anderson was in Japan with his wife Joyce and daughter Susan. | Source: Courtesy of Hiroto Kono and the Kubo family.
At the Tokyo conference, Anderson asked questions in five or six keynote sessions, and he found himself communicating very well with these top theoretical physicists, Felix Bloch, Lars Onsager, and Nevill Mott. Anderson's own speech on contemporary magnetic research was followed by Kubo and other young Japanese physicists, which gave him a boost of confidence. On the way home, he realized that he was no longer just a novice researcher in solid state physics, but a researcher with enough ability and research taste to independently carry out theoretical physics research.
During the first 15 years at Bell Labs, the papers he wrote independently were largely a perfect combination of intuition and detailed calculations. These include the Bardeen-Cooper-Schrieffer (BCS) model, which integrates the Coulomb effect into the phenomenon of superconductivity, and two other papers that won him the 1977 Nobel Prize in Physics.
What caught the attention of the Nobel prize judges was a strange phenomenon, Anderson found that waves that would otherwise propagate normally could be confined to an area by a disordered medium. George Feher, a colleague who works at Bell Labs, collected a large amount of spin resonance data in polysilicon, and from this seemingly complex data, Anderson constructed a simple model of the behavior of electrons in a disordered lattice. He hypothesized and went on to prove that the quantum tunneling effect in this disordered lattice would be severely suppressed, so that electrons that could have freely traveled between the ordered lattices would be constrained in this disordered lattice. Like spontaneous symmetry breaking, disorder causes the wave function to be constrained within a local region, a phenomenon now known as Anderson localization.
Another paper that the Nobel Prize jury focused on was Anderson's study of a molecular magnetic moment embedded in a non-magnetic metal with unpaired spins. Anderson obtained the data from colleague Bernd Matthias and spent weeks studying it carefully. The final paper on magnetic moment analysis was one of the best of all his papers. He summarized the experiments and discussed the previous theories of the subject, and then he gave a model of Hamiltonian quantities, first qualitatively discussed the special cases, and then analyzed them using the Hartree–Fock method, from which important conclusions were drawn, and finally gave his estimated limits.
Anderson likes to chat with experimental researchers, and always shows a strong curiosity and curiosity about the technical details involved in experiments. He spent a lot of time understanding the motivations and strategies of the experiment, and enjoyed the satisfaction of getting the first-hand experiment data by hand. In a 1999 oral history interview organized by the American Physical Society, he described himself as "sixty percent theoretical physicists and 40 percent experimental physicists," though he never actually completed an experiment himself.
3. Cambridge
Between 1961 and 1962, Anderson used his sabbatical year to make a brief visit to Cambridge University for a year, during which time he published only a short paper, but his influence led to the nobel prize of two other physicists. The first was Brian Josephson, who learned about symmetry breakage from Anderson's graduate class. After class, Josephson and Anderson discussed for hours the meaning of the phases of the microscopic wave functions of superconductivity, and less than a year later, Josephson published a short paper predicting the DC and AC Josephson effects, a work that earned Josephson the Nobel Prize in Physics in 1973.
For Peter Higgs, who won the Nobel Prize in 2013, Anderson played a similar role. While sipping tea with a particle physicist at Cambridge, Anderson heard that existing gauge field theories could not give masses to particles carrying weak forces in the nucleus of atoms. He suddenly found that if some variable substitutions were made, the Coulomb effect in the BCS superconductivity model he had studied earlier had exactly the same effect as the mass of elementary particles. In 1963, Anderson published a related article in the Physical Review. Higgs was acutely aware that the relativistic version of Anderson's thinking was exactly the answer needed.
At the end of the sabbatical year, Both Anderson and his wife developed a crush on Britain, so Anderson happily accepted an invitation from Mott, head of the Physics Department at Cambridge, to spend half his time teaching in the Solid State Physics Theory Group. Bell Labs also agreed that Anderson would spend only half of his time working on the lab. From 1967 to 1975, such an agenda led him to teach and mentor postgraduate students at Cambridge from October to March. Some of the work he did during this period is listed in figure 4.
Figure 4. Timeline of some of Anderson's research activities (this list is not complete).
An important contribution of Anderson was the explanation of the Kondo effect. The Kondo effect refers to a non-magnetic metal doped with magnetic impurities whose resistance will appear at very low temperatures at a minimum value in the trend line of temperature changes. To explain this phenomenon, a theoretical model must be constructed that can characterize the spin configuration near the ground state, and the model needs to exhibit this abnormal trend change law under low temperature conditions. This problem was the most challenging electronic multibody problem of the 60s. Anderson found the answer in 1970. At first he completed a thesis full of formulas with two junior collaborators, and then he completed a more outlining and elegant thesis on his own. In both papers, it was discovered that the reintegrate group method was invented and applied a full year before Kenneth Wilson.
A few years later, Anderson and the illustrious Welsh physicist Sam Edwards came up with a model that describes the magnetic behavior in heterometallic alloys, which is today's famous spin glass. Their solution to the spin ground state was only an estimate at the time, but in an attempt to optimize the results, they soon found that disorder and conflicting constraints appeared at the same time, implying that the amount of computation for the solution would exponentially increase with the number of spins in the system.
The same computational explosion problem also appears in many areas outside of physics, such as the famous salesman best route problem. Although difficult to calculate precisely, Anderson's model has been loved for many years. Because what his model can describe is beyond the scope of physics, with a slight change in variables, it can be applied to flight schedules, pattern recognition, integrated circuit design, communication coding...
4. More is different
In 1972, Anderson published an article titled "More is different: Broken symmetry and the nature of the hierarchical structure of science." The article unequivocally refutes a prejudice that has long pervaded the physics community. Many physicists working in high-energy physics will consciously or unconsciously consider their field to be more fundamental and important, while those in solid and condensed matter physics are relatively less important. This bias is reflected not only in the prestige and reputation of scholars, but also in the allocation of specific research funds and resources.
Anderson acknowledges the partial reductionist view that everything we see in nature must be made up of the properties of elementary particles. What he denies is the idea that the complex behavior of multiparty systems can be derived directly from the properties of simple particles. On the contrary, nature has fundamental laws and laws waiting for us to discover at different scales, and those laws that exist only on the macroscopic scale are no less fundamental than the physical laws on the scale of elementary particles. Mechanisms such as symmetry-breaking, if you focus only on the nanoscale, can hardly find the reason for its emergence.
Anderson's emerging ideas in "More is different" have resonated not only in the field of condensed matter physics, but also among chemists, physiologists, economists, and "macroscopic" biologists who have been marginalized by molecular biologists, they have begun to look for and emphasize the fundamental core of their fields of expertise. Another in Ying and Anderson's article further argues that when the system becomes large enough, one should no longer think about the reduction of symmetry, but rather about the increase in complexity.
Ten years later, Anderson and a number of scientists founded the Santa Fe Institute, an institution focused on the study of complex systems. Bringing together experts from the fields of economics, neuroscience, computer science, etc., it has become a fertile ground for the study of complex and nonlinear dynamics.
5. Princeton
In 1975, Anderson replaced half of his teaching position at Cambridge with half of his teaching position at Princeton University. His teaching style was still the same as when he was at Cambridge, often appearing to be less systematic and orderly, but fortunately he was allowed to teach as he pleased. After almost 10 years of teaching, he finally wrote a classic textbook in the field of condensed matter, Basic Notions of Condensed Matter Physics. With the publication of the book, he officially retired from Bell Labs, officially changing Princeton's half-time teaching position to a full-time teaching position.
Anderson also maintained a consistent style of research at Princeton: to root theoretical research in experimental data; to look for "anomalies" that found that experimental data did not match current theory; and to construct a model that would highlight the physical content of Hamiltonian quantities. His extraordinary intuition often helped him find answers directly, but he increasingly relied on others for the technical details of mathematics. So he recruited three colleagues and urged them to build a scale theory on the localization of disordered induced waves.
With the joint efforts of the four people, the result was not only elegantly reproduced and the wave localization result previously proposed by Anderson, but also generalized this result from the original 3-dimensional to 2-dimensional and 1-dimensional situations.
Being a graduate student at Anderson wasn't easy because he almost never taught instructions on how to get started with computing. More than one or two students likened his approach to mentoring graduate students to an "oracle." Students often leave the conference room confused after meetings with him, not understanding what he is trying to say, until weeks or even months later. The Russian physicist Anatoly Larkin once said, "God speaks to us through Anderson, and it is inconceivable why God chose such an incomprehensible spokesman." ”[11]
6. Superconducting large accelerator SSC
In 1970, Anderson learned on the bulletin board of a meeting of the American Physical Society that the budget for building the National Accelerator Laboratory (later Fermilab, the world's second-highest-energy particle accelerator, after CERN's Large Hadron Collider) could threaten funding for other "small science" projects across the country. He then wrote an article in the journal New Scientist opposing the plan, criticizing the "big science" practiced in the high-energy physics community. Years later, he again became an opponent of the Superconducting Supermassive Accelerator (SSC), a giant machine built in the United States to test the Standard Model of particle physics.
On August 4, 1993, Anderson and theoretical physicist Steven Weinberg (died July 23, 2021), one of the main founders of the Standard Model, were questioned back-to-back about accelerator construction projects at a hearing. Weinberg's defense of accelerator projects has always revolved around the fundamentals of the work, and Anderson argues that whether the Standard Model is proven or falsified is not worth paying an undue price, especially at the expense of funding for other research areas that are of equal value, or even have more practical value in many areas. Although a large sum of money had already been invested in the accelerator project at the time, the project was halted two months after the hearing.
Historians of science generally agree that the eventual demise of the SSC project has little to do with debate and rhetoric among scientists. The real reason why the government and parliament chose to abandon it should be blamed on the project's ever-increasing cost budget, poor project management, and the trade-offs of politicians.
7. High temperature superconductivity
In 1986, a major earthquake occurred in the entire field of condensed matter physics, and the superconductivity phenomenon of copper oxide ceramic materials appeared under unexpected high temperature conditions. Anderson has long been interested in superconductivity research, and he was the first theoretical physicist to propose an explanation for new superconducting phenomena. His theory is extremely subversive, completely abandoning the electron-photon interaction mechanism used to explain traditional alloy superconductivity, and instead emphasizing short-range Coulomb repulsion between electrons.
In his paper, Anderson suggests that the study of oxide superconductivity should use the ferromagnetic model proposed by John Hubbard a few years ago. Incidentally, Anderson often claims to be the inventor of the Hubbard model, which is basically and indeed true. The exact solution of the Hubbard model is still unknown, and Anderson roughly guessed the boundary range of the solution, and its ground-state multibody wave function is very similar to the electronic state of the resonant valent bond studied by Anderson and colleague Pauling 40 years ago.
In March 1987, the American Physical Society held a prestigious conference on the topic of high-temperature superconductivity, and Anderson was the first physicist to speak at the meeting. Not only did he spend the night discussing with the scholars, he was the only theoretical physicist to attend the press conference, responsible for briefing the public at the end of the meeting the next morning. Other theoretical physicists had different ideas about newly discovered superconductors, and for the next 20 years, Anderson was unable to get most of his colleagues to accept his views, largely because his own views changed with the results of the newly discovered experiments.
Anderson was a hot-tempered and competitive man who had good relations with almost all experimental physicists, but in debates with theoretical physicists, it was easy to speak fiercely. Unfortunately, his attitude toward the theory of high-temperature superconductivity is mixed with too many personal emotions, and although his own ideas are often changing, he cannot tolerate other theoretical researchers having different opinions and even being unable to work with them.
Some will respond in the same way, and the field has gradually become a fiery fighting arena, which makes many young people reluctant to join. Today, the hostility of the early years has dissipated, and no single theory can explain all oxide superconductivity. Perhaps the only widely accepted one is the view that Anderson once embraced so much: that subtle and unpredictable senses may be at the heart of the problem of multibody physics.
8. A life without regrets
In his later years, Anderson often interacted with listeners or readers outside of physics circles. He would publish book reviews in journals and magazines that were not limited to physics, not even science, and he would talk about military scale control, complexity, religion, science politics, futurology, clash of cultures, the meaning of science. He thinks about the philosophy of science because he recognizes that the structure of science is more like an interlaced web than a hierarchical evolutionary tree or pyramid. In his 1994 essay for the British Newspaper The Daily Telegraph, he said that everyone should know the following four things about science: science is not democracy; computers cannot replace scientists; statistics are sometimes misused and often misunderstood; and good science has aesthetic qualities.
The second point reflects Anderson's subtle sense of the use of computers in theoretical physics, on the one hand, his envy for the computational work of friends such as William McMillan and Volker Heine, and on the other hand, he despises the creative help that computers can do more than other scientists of his generation. This tendency, for example, led him to despise the numerical calculation of the electronic structure of matter and to be too lazy to familiarize himself with the current state of the discipline. Ironically, almost all of the important recent advances in high-temperature superconductivity have come from computer simulations of hubbard models and their variants.
Anderson loves knowledge, reason, culture and nature. In addition to physics, he was passionate about sports, politics, gardening, Go, Romanesque architecture, and many other things. His friends around him know him as warm, generous, and loyal, especially when others need him, and he has helped frustrated students or postdocs get back to normal life more than once. He's good at telling stories, but not at telling jokes. A few years after winning the Nobel Prize, he once wore black sunglasses and a fake beard and attended an academic conference under a pseudonym, where 10 percent of the speech topics carried the words "Anderson model."
Anderson's wife, Joyce, played an integral role in his career, especially during his time at Bell Labs, where Heyce's demeanor and appearance gave the impression of a rising star under Joyce's meticulous care. Joyce will also use his English major skills to help Anderson polish the manuscript. In 70 years of marriage, Anderson rarely stayed in the office after 5:00 p.m., knowing that his wife at home was waiting for him to come home.
Philip Anderson is one of the brightest stars in the sky of theoretical physics, and his theoretical contributions have had a profound impact across a wide range of fields. Future historians will recognize him as one of the greatest scientists in the world.
Resources
[1] L. Plévert, Pierre-Gilles de Gennes: A Life in Science, World Scientific (2011), p. 287.
[2] A. Zangwill, A Mind Over Matter: Philip Anderson and the Physics of the Very Many, Oxford U. Press (2021).
[3] P. W. Anderson, Phys. Rev. 86, 694 (1952).
[4] P. W. Anderson, Phys. Rev. 109, 1492 (1958).
[5] P. W. Anderson, Phys. Rev. 124, 41 (1961).
[6] P. W. Anderson, Phys. Rev. 130, 439 (1963).
[7] P. W. Anderson, G. Yuval, D. R. Hamann, Phys. Rev. B 1, 4464 (1970); P. W. Anderson, J. Phys. C: Solid State Phys. 3, 2436 (1970).
[8] S. F. Edwards, P. W. Anderson, J. Phys. F: Met. Phys. 5, 965 (1975).
[9] P. W. Anderson, Science 177, 393 (1972).
[10] E. Abrahams et al., Phys. Rev. Lett. 42, 673 (1979).
[11] P. W. Anderson, New Sci. 51, 510 (1971).
[12] P. W. Anderson, Science 235, 1196 (1987).
[13] P. W. Anderson, More and Different: Notes from a Thoughtful Curmudgeon, World Scientific (2011).
[14] P. W. Anderson, Stud. Hist. Philos. Mod. Phys. 32, 487 (2001).
This article is reprinted with permission from the WeChat public account "Jizhi Club", translated from: Physics Today.
Original link:
https://physicstoday.scitation.org/doi/full/10.1063/PT.3.4960
Special mention
1. Enter the "Boutique Column" at the bottom menu of the "Return to Simplicity" WeChat public account to view the series of popular science articles on different topics.
2. "Return to Park" provides the function of retrieving articles on a monthly basis. Follow the official account, reply to the four-digit year + month, such as "1903", you can get the index of articles in March 2019, and so on.