Including Science and Nature, in a number of science news awards held at the end of 2021, the gene editing technology CRISPR was listed for the first time directly in human clinical trials and achieved initial efficacy.
The use of gene editing technology commonly known as "gene scissors" to treat human diseases, bringing people unlimited imagination space. So, how exactly will it be realized? What about the actual progress so far? We invited zhang Tiankan, a well-known popular science author, to talk about this matter.
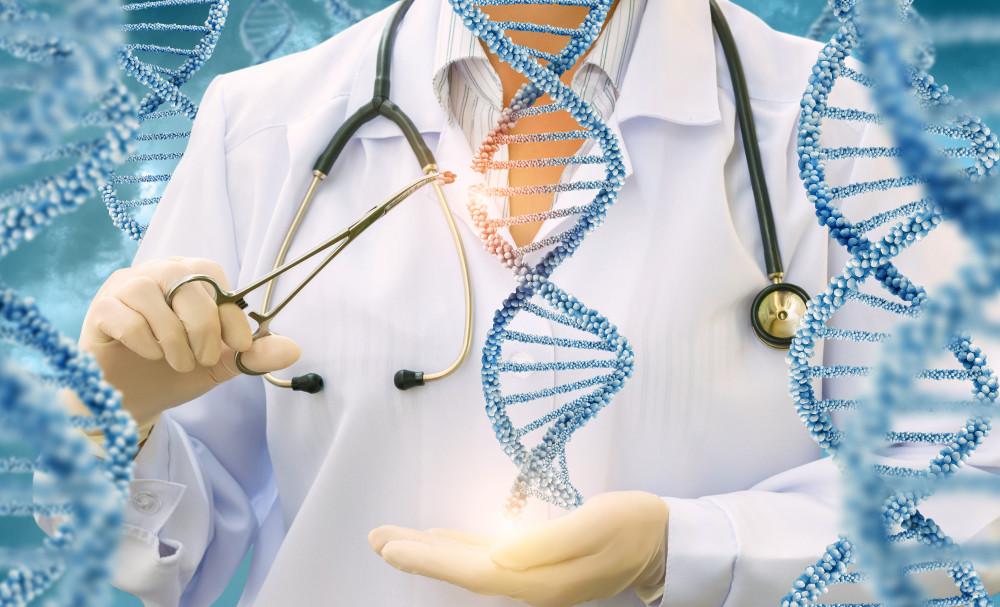
Why genetic scissors can cure diseases
As the nature magazine's 2021 Top 10 Science News presentation put it: "Since its inception, the gene-editing technology CRISPR has been seen as a rule-changer in the treatment of disease. But for this dream to come true, many diseases will require researchers to successfully implant CRISPR-Cas9 into humans and prove that it safely and efficiently edits the only target gene. ”
The gene scissor CRISPR-Cas was originally discovered in archaea by scientist Francisco Mojica of the University of Alicante in Spain, and later in most bacteria and in the vast majority of archaea's genomes.
CRISPR-Cas is a defense system used by bacteria to recognize and destroy the invasion of viruses, bacteriophages and other pathogens, and is an immune weapon produced by bacteria and viruses fighting each other in the evolution of life. In their natural state, viruses can integrate their genes into bacteria, using the cells of bacteria to copy their own genes. However, after bacteria evolved the CRISPR-Cas system, they could quietly cut the genes of the virus invading bacteria from their own genomes, which is actually a kind of genetic scissors that can cut off the invading genes.
Using the principle of cutting genes in the CRISPR-Cas9 system, researchers can design "gene scissors" that bind to the target sequence (target gene) on the genome and turn off or cut a certain target sequence gene. Thus, CRISPR-Cas9 can be used to delete, add, activate, or inhibit target genes in human, animal, and plant cells.
In 2013, CRISPR-Cas9 technology was first applied to gene editing in mammalian cells. In 2015, this technology was named the first of the top ten scientific and technological breakthroughs of 2015 by Science magazine.
For the treatment of disease, CRISPR-Cas9 can be used to target and modify the "typo" in the 3 billion letter sequences in the human genome, that is, the pathogenic gene, thus treating a variety of diseases, especially genetic diseases.
Now, CRISPR-Cas9 gene scissors have been applied to human cells, rats, mice, fruit flies, zebrafish, yeast, bacteria, plants and other gene editing. For humans, genomic function identification can be performed, and it can also be used to fight viruses, treat cancer and immune diseases.
In terms of cancer treatment, animal testing has now yielded important results. The CRISPR-Cas9 system not only targets knockout oncogenes, but also repairs mutated oncostatic genes under the action of exogenous DNA templates. In mice, it was found that the CRISPR-Cas9 system can target the cutting of non-small cell lung cancer-related oncogene EGFR, which can effectively inhibit the proliferation of non-small cell lung cancer cells and the growth of allogeneic transplant tumors in mice, prolonging the survival of mice.
In addition to treating blood diseases and cancer, the CRISPR-Cas9 system and other CRISPR systems can also treat eye diseases, AIDS, cystic fibrosis, muscular dystrophy, Huntington's disease, chronic infections (such as urinary tract infections), and even diabetes.
Where is the difficulty of genetic scissors
However, an important flaw in the CRISPR-Cas9 system is off-target, that is, while the target gene is removed, genes similar to the target gene or genes adjacent to the target gene can be cut off, which may bring serious side effects to patients.
Some researchers have found that when the CRISPR-Cas9 system acts on human cells, the average off-target rate of potential off-target sites is about 40%. An article published in the journal Nature Method in May 2017 stated that the CRISPR-Cas9 system introduces a large number of unpredictable mutations into the mouse genome, that is, large-scale off-target. However, follow-up investigations found that the large-scale off-target phenomenon was a misjudgment generated by the author's use of mouse controls with unclear genetic backgrounds, so the article was retracted.
Still, the off-target effects of the CRISPR-Cas9 system are a cause for concern. In response, the researchers explored why the CRISPR-Cas9 system has an off-target effect.
The CRISPR-Cas9 system is a defense mechanism that protects bacteria from viruses. When a virus invades a bacterium and stores a part of the gene in the bacterium, this built-in viral DNA plays a role in genetic memory. If the same virus attacks the bacteria (or its offspring) again, the bacteria quickly recognize the virus and dispatch the Cas9 protein to track it down. Cas9 finds the hostile viral DNA in the bacterial cell, and if it is found to match the viral RNA stored in the bacterial genome, it will cut the viral gene, thereby eliminating the threat of the virus invading again.
However, Cas9 sometimes guides the cutting of DNA or RNA sequences that are similar to the target DNA it is looking for, because it makes perfect sense for bacteria to cut these slightly different DNA sequences from an evolutionary perspective. Because the virus is constantly mutating, it can form a target gene that is slightly different from the target gene that Cas9 is looking for. By cutting slightly different DNA or RNA sequences, the CRISPR-Cas9 system is able to track the evolution of viruses and better protect bacteria from mutated viruses. This is one of the important reasons why the CRISPR-Cas9 system frequently goes off target.
However, the researchers believe that there are several ways to avoid the off-target of the CRISPR-Cas9 system in the future. First of all, the specificity of the guided sgRNA can be improved and the accuracy can be improved; the amount of Cas9-sgRNA can be reduced and controlled to avoid off-target; the Cas9 protein can also be modified to improve the specificity of the CRISPR-Cas9 system and reduce the off-target rate; the "off switch" of the Cas9 protein can also be used to avoid the ACCIDENTAL-Cas9 system from accidentally cutting normal DNA or RNA.
If these methods can be applied, and proven in practice that they can greatly reduce the off-target rate or zero off-target, CRISPR-Cas9 gene scissors may be truly used in the clinical treatment of diseases.
Experimental therapeutic effects offer hope
In June 2021, researchers at Intellia Pharmaceuticals and Regeneration Meta reported in the New England Journal of Medicine the results of a trial treatment for six patients with a rare disease called transthyroin amyloidosis, showing positive results.
Transthyroxine amyloidosis is a life-threatening disease in which misfolded transthyroxine protein is produced in the patient's main organs, such as nerves and the heart, and gradually accumulates, thus triggering neurological and cardiovascular symptoms, which in severe cases endanger the patient's life.
One drug the researchers developed, NTLA-2001, is actually a CRISPR-Cas9 gene scissors that can be targeted and persistently knocked out of misfolded transthyretin. On the 28th day after the patient's injection, the researchers observed that the patient produced a drug effect, showing that by targeting knockout of transthyroxine protein can permanently reduce serum transthyroxine protein concentrations, thereby treating transthyroxin protein amyloidosis. After administration, some patients experienced mild adverse events, but all patients did not experience serious adverse events.
Prior to that, in 2020, another scientific team in the United States used the drug CTX001, also based on CRISPR-Cas9 gene editing therapy, to trial treatments β thalassemia and severe sickle cell disease.
β thalassemia is caused by a mutation in the globin gene, which prevents heme from carrying oxygen efficiently. Sickle cell disease is a red blood cell that contains abnormal hemoglobin S. When red blood cells contain a large amount of hemoglobin S, they are easily deformed into sickle-shaped. Because sickle cells are stiff, it is difficult to pass through the capillaries, resulting in obstruction of blood flow, reducing the oxygen supply to tissues at the site of capillary obstruction. Obstruction of blood flow can cause pain and can prolong over time, can lead to damage to the spleen, kidneys, brain, bones, and other organs, and can also cause kidney failure and heart failure.
The researchers used CTX001 to genetically engineer the collected patient's stem cells in vitro and then transfuse them back into the patients. CTX001 can engineer a patient's hematopoietic stem cells into red blood cells that produce high levels of fetal hemoglobin (HbF), so that the patient can be oxygenated. HbF is an oxygen-carrying hemoglobin that occurs naturally at birth and is then converted to adult form of hemoglobin. Elevating HbF levels by CTX001 reduces the transfusion needs of patients with β thalassemia, while reducing suffering and debilitating sickle cell crisis in patients with severe sickle cell disease. Trials have demonstrated that CRISPR-Cas9 gene scissors have initial therapeutic efficacy β thalassemia and severe sickle cell disease.
The breakthroughs in these two trials have greatly encouraged people's confidence in using gene editing technology to treat human diseases. Of course, the two breakthroughs that have been made so far are still aimed at relatively rare niche diseases, and the efficacy still needs to be further verified. But this brings enlightenment and hope to gene-editing technology to treat common diseases that are more harmful to human beings.