Recently, a team of international researchers measured the lifespan of neutrons outside the nucleus with extraordinary precision. According to their measurements, the neutron survived for 14.629 min, or 877.75 sec.
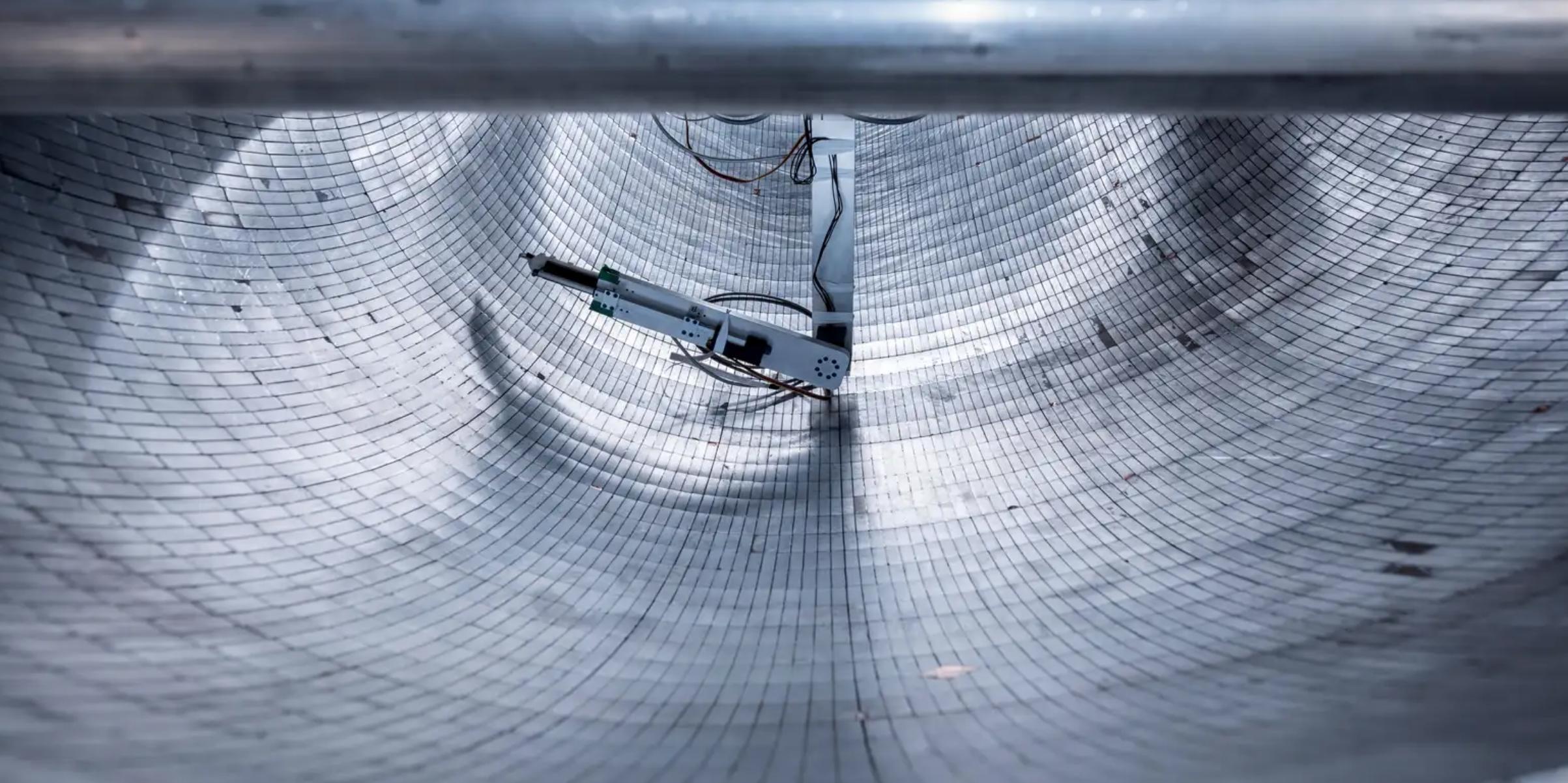
Above: A curved array measuring the life of neutrons in a UCNt instrument.
In fact, neutrons are not alive. However, particles (the components of atomic nuclei) do decay into positively charged protons and other particles. Neutrons outside atoms are called free neutrons, and the timing of their decay is very important for physicists who are trying to understand how many neutrons in the very early universe could form the first atomic nucleus, and different numbers of neutrons would produce different numbers of elements that we find in the universe today, and these elements gave rise to life.
Scientists have known for years that a lone neutron will die (decay) in about 15 minutes, dating back to the original measurement of the decay of free neutrons in 1951. But the new measurement work (the result of years of experiments with the UNCtau instrument at the Los Alamos Neutron Science Center) is the most accurate of its kind. The team's findings were published last week in the Physical Review Letters.
Shannon Hoogerheide, a physicist at the National Institute of Standards and Technology, said the paper was "a very impressive result." The UCNtau team did a good job of carefully dealing with a range of system uncertainties in the experiment, and independent blind analysis added confidence to the results. This result is an important step in improving Standard Model testing. ”
Brad Filippone, a physicist at the California Institute of Technology who led the study, said: "This finding provides an independent assessment to solve the mystery of neutron lifespan. If combined with other precise measurements, this result could provide a wealth of search evidence for the discovery of new physics. ”
Above: Neutron counting master detector for the UCNt experiment at the Neutron Science Center.
Of course, this is not the final conclusion of the lifespan of free neutrons. It is also unlikely to be conclusive. In fact, there are two long-standing methods for measuring neutron life. One is the beam method, which observes a beam of neutrons and calculates the number of protons that occur when the neutron decays. The other is the bottle method, which traps free neutrons in a large, cold, magnetized bowl (or bottle) and counts the number of remaining neutrons.
"The bottle experiment measures survivors, the beam experiment measures the dead," explains Geoff Greene, a physicist at the University of Tennessee and oak ridge National Laboratory. Beam experiments, on the other hand, sound hard, and actually really hard. ”
The beam method reliably produces results about 9 seconds longer than the bottle method; the difference is large enough that the error bars of the two methods do not overlap. Physicists don't know if this mismatch is a sign of new physics that no one understands yet.
"If you're not paranoid, you shouldn't be doing this kind of work."
Because the bottle method measures surviving neutrons, it ignores by definition anything that happens when neutrons decay. Beam experiments, on the other hand, are dedicated to measuring decay products (in this case, protons). If new physics were at work, it could mean that in addition to β decay, neutrons decayed through other ways (what physicists call "channels"), producing particles other than protons that are still hypothetical: axions, dark matter, WIMPS — whatever you choose.
Physicist Jeff Green explains: "The allure of this approach is that it can predict that the bottle method will have a lower lifespan than the bundle method because there is another channel. ”
If the answer to this discrepancy is indeed new physics, the experimenters of the beam and bottle methods will only know this after repeatedly proving that their respective experiments have no uncertainties and that no greater systematic errors are at work.
We're still a long way from being known; most physicists aren't the kind of people who would cry wolf, and if anyone really thinks this difference in lifespan is evidence that neutrons can decay in other ways, they have to make sure that this is consistently proven in all other experiments involving nuclear β decay.
For now, physicists are still trying to study the error bars of the beam and bottle methods, trying to figure out what the results tell them. Once the water is lost, scientists may be able to determine what caused the 9-second gap. This new experiment is the most accurate measurement done with the bottle method for several reasons.
Another scientist who led the study, Daniel Salvat, a physicist from Indiana University Bloomington, said: "The difference between our technique and other 'bottle' measurement methods is that we are both a magnetic neutron trap and a neutron detected in this magnetic neutron trap. This result uses a completely new data set that is completely different from our previous results, and the data is blind, so we will not be swayed by the previous less precise results. ”
Above: Indiana University student Bailey-Ann Slaughter in a neutron trap.
The team's final calculation of neutron decay was an unweighted mean of three independent blind analyses. "Blindness is a good technology," Green said, noting that if there is some kind of systemic effect, such as a hole in a bottle, then blinding is useless. In addition to decay, the team can also lose neutrons in another way.
Green said the beam method experiments have produced very consistent results because they have become more precise. Previous bottle measurements of this cold neutron have produced a slightly longer lifetime, while the magnetic gravitational trap used by the recent research team has made the neutron's lifetime slightly shorter.
Ultracool neutrons are used to help us understand how long they last at the beginning of the universe's unusually hot heat, which is a bit counterintuitive. At first, the light element was formed by a soup of raw, overheated particles. This process, known as big bang nucleosynthesis, occurs on different time scales, depending on how long the free neutrons stay.
In the first few microseconds of the universe, it was so hot that there were no protons, neutrons, or nuclei, but a "quark soup," and shortly thereafter, the quarks condensed into droplets, known as protons and neutrons.
After a few seconds, the universe begins to cool, and protons and neutrons can stick together to form the light elements hydrogen, helium, and lithium. If neutrons had a short lifespan, all neutrons would quickly become protons, and in the early universe we would only get hydrogen. But that's not what the team observed — neutrons have a lifespan closer to 15 minutes.
In addition to the speed of the early universe, the lifetimes of neutrons also had a profound impact on physicists' exploration of dark matter, solar fusion, and the Standard Model of Physics. But to get a handle on all of this stuff, researchers must first figure out why these neutrons seem to decay at different times.
If friends like it, please pay attention to "Knowing the New"!