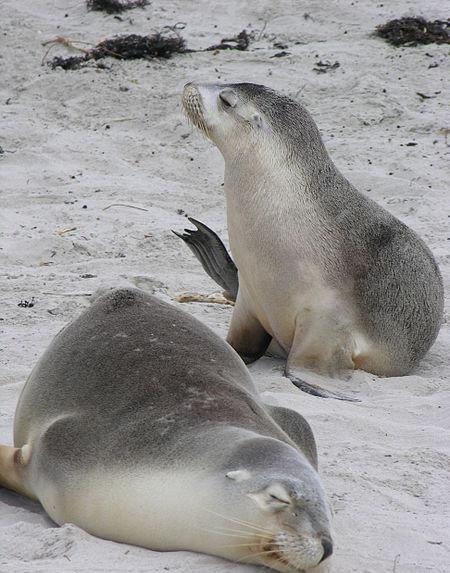
The feeding habits of Australian sea lions in the wild were analyzed by vomit and stomach samples
Rebecca R. McIntosh A, Brad Page B and Simon D. Goldsworthy B
A Sea Mammal Ecology Group, Zoology Department, La Trobe University, Bundoora, Vic. 3086, Australia.
B SARDI Aquatic Sciences, 2 Hamra Avenue, West Beach, Adelaide, SA 5024, Australia.
Summary: Food scraps extracted from the digestive tract and vomit of Australian sea lions (Kangaroo Island, South Australia) in Seal Bay (Kangaroo Island, South Australia) to identify predatory food species. Four of the 8 digestive tract samples collected (50%) contained only prey. On the basis of the biomass reconstruction of cephalopod food residues, octopus accounted for 40% of the sample biomass, Sepia apama (sepia apama) accounted for 30%, and softfish accounted for 14%. Several fish remains were found in the sample: Monocanthidae, Platycephalus sp., Centroberyx lineatus, Pempheris multiradiata, Sillago flindersi, Trachurus novaezelandiae. In addition, carapace fragments of Jasus edwardsii and Ovalipes australiensis, feathers and bones of Eudyptula minor, and shark ovary sheaths (egg species and cat sharks) have been found.
Brief introduction
Australian sea lions (Neophoca cinerea) range from Kangaroo Island (Kangaroo Island) in South Australia to the Horteman Abrojos Islands in Western Australia (Gales et al. 1994). It is a sea lion that lives only in Australia, with a total number of approximately 9794-12000 head (Gales et al. 1994; Goldsworthy et al. 2003)。 The sixth largest breeding site in South Australia is Seal Bay (35°41′S, 136°53′E) and the fourth largest breeding site is on Kangaroo Island, where about 233 pups were laid during the 2003 breeding season (95% confidence interval 219–247) (McIntosh et al. 2006).
Due to the strong seasonal cold water upwelling zone in the sea south of Kangaroo Island, its fertility is classified as moderate by global standards (Ward et al. 2004). New Zealand fur seals (Arctocephalus forsteri) (Shaughnessy et al. 2005) with a higher density (15,800 juveniles) in South Australia and up to 80% of the total Australian sea lion cubs (1994 juveniles) (Goldsworthy et al. 2003; McKenzie et al. 2005)。 In addition to the absence of seasonal reproductive cycles, Australian sea lions have a gestation period of 14 months, a estrus season of 5-9 months, and a lactation period of at least 15 months (Higgens 1993; Higgins and Gass 1993; Gales et al. 1997)。 For long-lasting breeding, the species makes full use of food sources in this breeding area, including seasonal and continuously available foods.
Limited analysis of stomach contents and feces has determined the feeding habits of Australian sea lions, including teleost fish, squid, squid cuttlefish, octopus, sharks (including Australian tiger shark Port Jackson sharks), rock lobster rock lobsters, small crustaceans and penguins (Marlow 1975; Gales and Cheal 1992)。 Adult female Australian sea lions in Seal Bay dive continuously in the sea, with an average depth of 41.5-83.1 m, of which 35% of the time is spent at 20% of their deepest diving depth (Costa and Gales 2003). Based on these findings, the Australian sea lion has been described as an opportunistic benthic forager (Richardson and Gales 1987; Gales and Cheal 1992; Ling 1992; Costa and Gales 2003)。
Assessing the Australian sea lion diet by analyzing hard objects in the feces is useless. Gales and Cheal (1992) found that there were few hard objects in Australian sea lions that could recognize feeding habits. Feeding experiments on captive Australian sea lions have shown that less than 2% of otoliths are removed after passing through the digestive tract, while the removal rate of cephalopod beaks in the excrement varies widely and is biased toward smaller prey (Richardson and Gales 1987; Gales and Cheal 1992)。 This may be due to the presence of gastrolaria, which may grind and destroy hard objects, or leave hard objects trapped in the stomach (Needham 1997). In the sea lion family, the proportion of cephalopod hard parts in stomach samples is often greater than that of smaller hard parts in fish, which makes food study of these sample types biased over cephalopods (Gales et al. 1993; Lalas 1997)。
The study aimed to analyse the vomit and digestive tract of Australian sea lions in Seal Bay to determine their primary feeding habits based on the hard parts found in the sample. The presence and size of gastrolithia have also been studied.
Research methodology
This study was conducted at the Australian Sea Lion Habitat at Seal Bay Nature Conservation Park, Kangaroo Island, South Australia (Fig. 1). Samples collected during habitat surveys conducted in the main habitat and western habitats between August 2002 and March 2005. The eastern part of the habitat is a no-navigation zone and no survey has been conducted. Each survey takes a day on foot. All surveys started in the western part of the habitat, with 1-3 investigators crossing the habitat to reach the eastern end of the main habitat. During the breeding season (December 2002–August 2003, June 2004 –December 2004), the survey was conducted 4–7 days a week. Outside of the breeding season, a one-day survey is used each month as part of the monthly colony count. A total of 324 surveys were conducted during the study period.
In habitat surveys, all sea lions are observed and it is easy to find dead individuals. All dead sea lions found in the survey were sex-identified, grouping (adult or juvenile) was recorded, and standard body length (nose to tail), body circumference, and weight (if possible) were measured before removal of the digestive tract. Adult sea lions are distinguished from juvenile sea lions based on the degree of development of their reproductive organs.
Vomit is collected at the right time when sea lions are observed to be sick to their stomachs or when fresh and unpassed vomit is found near sea lions. Only fresh vomit is collected to maximize the variety of prey, as prey is likely to dry out, decompose, or disperse over time (Tate 1981).
Separately load the vomit and digestive tract contents into containers, label and freeze at -20 °C, or store in 70% ethanol on the spot, which is then sorted and analyzed. Food scraps are sorted by rinsing hard matter with 0.5 mm and 1 mm sieves. Otoliths, fish bones, penguin bones and feathers, crustacean shells and eye crystals are dried and preserved, and shark egg sheaths and cephalopod beaks are stored in 70% ethanol. The gastrolar is washed and dried before weighing and then stored separately from other hard parts. The rock type was identified by Dr John Webb (Department of Earth Sciences, La Trobe University, Bundoora, Victoria), and the gastrolars were weighed separately to report the total and average weight of each stomach.
By and reference specimens (Shark Sheath: Dr Kate Rodda, SARDI, Port Lincoln, South Australia; Crustacean crustaceans: Dr Gary Poore, Melbourne Museum, Victoria), Atlas of Cephalopod Beaks and Fish Otoliths (Smale et al. 1995; Lu and Ickeringill 2002) compared all prey residues into the least taxa. Prey is then classified and counted to obtain a minimum number of individual prey per taxonomy.
Dietary taxa may be biased toward cephalopods in the stomach and vomit, so only the cephalopod components in food are quantified. A well-preserved cephalopod beak is measured using a digital caliper (accurate to 0.01 mm) for the regression equation to estimate prey mass. The length of the squid beak is judged by the length of the upper and lower beaks, and the length of the upper and lower mantle membranes is used for octopus and squid. The relative importance of each cephalopod prey taxon was assessed using three standard methods, including the percentage of abundance (the percentage of the total cephalopod prey populations constituted by each cephalopod prey taxon) and the percentage of occurrence frequency (the percentage of sample containing a given cephalopod prey taxon). However, due to the importance of bait taxa in dietary studies, body data (e.g. Hyslop 1980) best reflect the importance of dietary studies, so the most appropriate assessment is based on the percentage of biomass allocation (within the area or volume of the individual body).
Using the relationship between beak measurements and cephalopod masses, the regression equation is used to estimate the mass of cephalopod prey (Table 1). Several regression methods used to estimate prey biomass based on beak morphological measurements are based on data from other species, and/or the regression methods used do not include predicting a small subset of individuals with a longer or shorter beak length. Therefore, biomass reconstruction should be interpreted with caution. Estimate the minimum number of cephalopods in each sample by counting the number of upper and lower beaks. Samples containing fewer confirmed beaks than unidentified debris, such as lens and beak fragments, were recorded as containing the least number of unidentified individuals.
Biomass estimation is based on the minimum number of individuals per sample, using the upper and lower beaks. The percentage of biomass for each prey is calculated by adding the percentage of upper and lower beak biomass for each prey (Table 1).
outcome
A total of 16 ruminants and 8 digestive tracts were collected during the study period. Of the 8 digestive tracts, 4 contain only gastrolaria and 4 contain gastrolaria and prey remains (Table 2); they all contain the beaks of cephalopods, a hard part containing crustaceans, and a hard part containing fish. No hard parts are found in the intestines. Not all ruminant samples can be attributed to specific age/sex categories of sea lions. Prey was detected in all 16 ruminant specimens, including 15 cephalopods and only 1 hard part of crustaceans. Eight ruminant samples contained hard parts of the fish. A total of six cephalopod species (Table 3), six fish species, two crustaceans, two shark egg sheaths and one bird species (Table 4) were identified.
A total of 125 cephalopod prey individuals were identified from 20 samples, of which 105 were determined and used for biomass reconstruction (Table 1). The most important prey (with a biomass of more than 10%) are octopus, giant squid and softfish in addition to the king squid (Table 3).
Of the specimens containing cephalopods, 7 samples contained only 1 cepod taxon, 8 samples contained 2 cephalopod taxa, 3 samples contained 3 cephalopod taxa, and 2 samples contained 4 cephalopod taxa. Of the seven samples with only one cephalopod taxa, three of them also contained fish hard bodies, shark egg sheaths, Eudyptula minor feathers, or carapace fragments from crustaceans. Contained 20 octopus individuals (weight range 11.4–98.0 g; average weight = 84 g, standard deviation s.d. = 162.2; average length = 36.3 mm, s.d. = 25.4; no other prey taxa were found in this sample.
The two most abundant prey species are octopus and giant squid, which also appear frequently (Table 3). The size of octopus varies greatly, and the size of giant squid varies less (Table 3). The minimum octopus sizes are 2.3 g and 11.4 mm, and the maximum octopus sizes are 7859.9 g and 245.4 mm, respectively. The smallest giant squid is estimated at 607.1 g and 198.4 mm, and the largest is estimated at 1775.7 g and 270.2 mm. Other species of squid are smaller than giant squid and contribute the least to the cephalopod biomass they eat, but are more abundant and occur more frequently (Table 3).
In softfish, only larger individuals can be classified, and they are all notodarus gouldii (Table 3). Compared with the arrow squid, other species-denoticable softfish account for a larger proportion of reconstructed biomass and abundance. The smallest individual sizes for other softfish are 2.9 g and 72.1 mm, and the maximum individual sizes are 773.6 g and 302.1 mm, respectively. Sepioteuthis australis, although large in average size, is not numerous and therefore has a low proportion of biomass (Table 3).
Fish ear stones and fish eyes or bones were found in 7 ruminants and 2 stomachs. The presence of vertebral process was used to identify Monocanthidae in three samples, but it was not possible to determine the type of consumption and the number of individuals consumed. Otoliths in ruminants are used to identify (Centroberyx lineatus), (Pempheris multiradiata), (Sillago flindersi), (Neoplatycephalus sp.) and (Trachurus novaezelandiae). The sample size of ichthyorites was small (Table 4) and many of the otoliths were eroded too badly to reconstruct the length and size of the fish assessed by measuring otoliths. The feathers of the little penguin were found in a ruminant. Carapace fragments of the South Rock Lobster (Jasus Edwardsii) were found in 3 ruminants and 1 stomach. A pike crab (Ovalipes australiensis) was found in the stomach of a dead young sea lion. Six cases of shark egg sheaths were found in 3 ruminants. Five of the egg sheaths are from the family Scyliorhinidae and the sixth is from an egg species.
Gastroliths (Table 2) were found in the stomachs of all 8 sea lions, consisting of metamorphic sandstone from the Kanmantoo Group (Drexel and Preiss 1995). The gastrolaria of the sub-adult (n = 2) (average 30.9 g ± 14 g, n = 3 gastroliths) are smaller than the adult females (n = 6) (average 95.5 ± 49.3 g, n = 25 gastroliths). The sample size is too small to make a statistical comparison.
discuss
This is the first study to quantitatively estimate the biomass and composition percentage of cephalopod prey preyed by Australian sea lions. Although stomach samples have been examined before (Marlow 1975; Gales and Cheal, 1992; Kemper and Gibbs 1997; S. E. Gibbs, Macquarie University, unpublished data), but this is the only study to analyze the ruminants of free-living Australian sea lions.
Quantitative analysis only analyzed the cephalopod components in the diet, as stomach samples and ruminants were biased toward cephalopod beaks and other large prey, thus providing a positively biased cephalopod number in the diet (Gales and Cheal 1992; Gales et al. 1993; Lalas 1997)。 For the Phocarctos hookeri of new Zealand's Otago Peninsula, more than a third of the colonies of chapter fish and the hard-shelled parts of most crustaceans and arrow squid are provided in ruminants compared to prey identified in fecal scats (Lalas 1997). Ruminant and gastric contents analysis may provide information about the cephalopod components in the diet, but less information about fish populations.
There are inherent biases in any dietary analysis method (Gales et al. 1993; Fea and Harcourt 1997; Lake 1997; Hume et al. 2004) and several questions, in particular the analysis of ruminants and the digestive tract of dead individuals. Dead individuals caught by trawling are likely to distort the diet of healthy individuals, as disease and poor physical condition may affect the type of prey that can be eaten, as previously suggested for cetaceans (De Pierrepont et al. 2005). Although there were no significant differences in the gastric contents of Otaria flavescens of dead, stranded individuals in South American sea lions and those of entangled healthy individuals (Koen Alonso et al. 2000), gastric samples from several other seal death individuals were often found to be empty (Pierce et al. 1991; Gales and Cheal 1992)。 Pierce et al. (1991) 61% of the stomach specimens of dead seals were found to be empty, but on examination of the entire digestive tract, 94% of the specimens contained the hard part of the prey. This is not the case in this study, as there is no hardware part in the gut.
Food debris in gastric contents and ruminants may be biased towards the type of prey, with the hard part of it remaining in the stomach for a longer period of time (Harwood and Croxall 1988; Pierce et al. 1991)。 Ruminants also contain other inherent biases in food analysis, as larger prey may be over-described (Gales and Cheal 1992; Gales et al. 1993; Needham 1997)。 It is estimated that captive Australian sea lion food passes through the digestive tract at an average rate of 14.9 h (Bodley et al. 1999) and large experimental markers (>1.2 mm in diameter) remain for more than 6 days (Richardson and Gales 1987). Because when sea lions are at sea, the remains of prey can be excreted, which can lead to ruminants and over-presentation in the stomach of prey taxa consumed at the last meal before sea lions are towed out of the ocean.
Cephalopods and other prey species contribute to the composition of the diet
The prey found in this study is similar to that found in previous Australian sea lion diet studies. Gales and Cheal (1992) examined 5 stomachs and 46 feces of free-range Australian sea lions. In the feces, they identified fish wreckage, octopus, squid, squid, lobster, patchy feet, shrimp, crabs, shark fragments and a feather. Cephalopod remains are found in only 24% of feces, including the beak of a small octopus and a portion of squid. The stomach (one of which is empty) contains a large octopus beak, a squid beak, and a shark egg sheath. Gales and Cheal (1992) also examined ruminants of one male and one female in a breeding trial of Australian sea lions; there were 26 octopus jaws in the ruminants of females, which represents a minimum recapture of 93% of octopuses fed by sea lions in that week's trial.
The current study of free-living Australian sea lion ruminants has found that there are more taxa in the diet, with 94% of ruminants containing cephalopod beaks and 50% of ruminants containing fish hard bodies. Half of the stomach samples contained cephalopod beaks, one of which also contained the remains of fish and the other of crustaceans. Six cephalopod species, six fish species, two crustaceans, two shark species, and one bird species were identified (Tables 3, 4). Using ruminants and gastric specimens, more prey can be identified because the hard part is retained in the stomach and less eroded than the small hard part in the feces (Gales and Cheal 1992).
Octopuses are the most abundant of cephalopods, accounting for the largest proportion of biological distribution (40%), but giant squids have the highest frequency (24%) (Table 3). There is some evidence that cephalopods are specifically depleted, with seven samples containing only one taxon of cephalopod prey, namely octopus, squid, or flea. This could mean feeding on aggregating cephalopods, or specific prey for individual sea lions. Three of the seven samples contained prey other than cephalopods, suggesting that individual sea lions are also opportunistic foragers.
The cephalopods found in this study are generally confined to shelf benthic fauna, but also include taxa that live in the open ocean. The continental slope (200 m deep) is located about 200 km south of Kangaroo Island. Adult female Australian sea lions forage on the continental shelf and dive to depths of up to 60–105 m (Costa and Gales 2003). Octopus, squid, arrow squid and Ley's pseudo-squid live at the bottom of the continental shelf (Stranks 1996; Uozumi 1998; Pecl 2001; Triantifillos et al. 2004; Jackson et al. 2005)。 Softfish (except for the arrow squid) are usually species that swim rapidly in open waters (Dunning and Wormuth 1998; O’Dor et al. 2002)。
Some cephalopods found in dietary samples of Australian sea lions are non-migratory and can often be found, such as the Leyser squid, whose populations are genetically isolated, suggesting that their juveniles or adults are rarely active (Triantifillos et al. 2004). However, dietary samples also contain highly dispersed prey, such as the giant squid of the southern coast, which consists of a genetic population (Triantifillos et al. 2004). In South Australia, giant squid gather to lay their eggs every year from May to August. Lifelong life history of one child (Arnould 1977; Wells 1977) makes this cephalopod possibly a seasonal abundance and predictable food source for Australian sea lions. Squid, the least abundant cephalopod in the Australian sea lion sample, may be a more difficult food source for Australian sea lions because both growth and spawning events vary with the environment (see Jackson and McGrath-Steer 2005 for a review).
Noncephalopod prey (Table 4) is predominantly benthic species (81%) that live in different habitats on the continental shelf. Southern rock lobster on rocky reefs from intertidal zone to 200 m depth (Edgar 1997) with high site selection certainties (Gardner et al. 2003; McGarvey 2003; Linnane et al. 2005)。 Pike crabs inhabit soft sandy bottoms in the subtidal zone (Jones and Morgan 1994). Cat sharks found in the waters of southern Australia are mostly benthic creatures that live in a variety of waters, from offshore to deep sea (Last and Stevens 1994). Australian sea lions are known to eat sharks and their egg sheaths (Marlow 1975; Gales and Cheal 1992)。 Judging from the appearance of the egg sheath cases in this study, the case of the cat shark egg sheath is eaten after laying, and the egg sheath case of the egg may be caused by eating a pregnant shark. Little penguins and Australian sea lions share a lot of common habitat, including the little penguins in the fur seal feeding habits of Kangaroo Island (Page et al. 2005). Most of the bony bait found in this study live in reefs and other benthic habitats (Table 4). Leatherjackets, flathead, eastern school whiting (depth 80m) and common bullseye are benthic creatures inhabiting reefs and seagrass beds on the continental shelf (May and Maxwell 1986; McKay 1992; Hindell et al. 2000; Jordan 2001, Shepherd and Brook 2003)。 Swallowtail is a benthopelagic benthopelagic that inhabits rocky reefs on the continental shelf and continental slope (May and Maxwell 1986); the yellowtail mackerel is a pelagic fish (to 150 m deep) and also coastal (Paxton et al. 1989).
Gastrolithic Gastroliths
The gastrolaria found in gastric specimens are all of the same rock type and are likely to have come from the same place. Compare this type of rock with rocks from Seal Bay (Pebble Beach, West No Sail Zone) and discover that this type of rock is distributed here. The role of gastrolithia in the stomach of the sea lion family is unknown. They may aid digestion (Needham 1997), but may also provide buoyancy control for marine tetrapods to facilitate underwater propulsion of the front fin (Taylor 1993). They are usually found in penguins and fur seals of the family Hippoceae, but not in walrusids, seals, or cetaceans (Taylor 1993).
May overlap with the diet of fur seals in the same region
In South Australia, the breeding ranges of Australian sea lions and New Zealand fur seals overlap. The eastern part of the Australian sea lion range also overlaps with the range of Australian fur seals, with a small number of migratory male sea lions on Kangaroo Island (Shaughnessy and Dennis 2001). The extent to which food competition overlaps between Australian sea lions and domain seals is unclear.
On Kangaroo Island, male Australian fur seals eat mostly benthic fish (96% of total dietary biomass), male New Zealand fur seals eat mainly birds (37% of total dietary biomass), and female New Zealand fur seals consume cephalopods (55% of total dietary biomass) (Page et al. 2005). Most of the prey of the Australian sea lions found in this study overlapped with the prey of kangaroo island domain fur seals.
Among the Australian sea lion samples in this study, octopus had the highest biomass (40%), but had a smaller proportion of cephalopod biomass in the fur seal diet (4% for adult female New Zealand fur seals; 5% for adult male New Zealand fur seals; 28% for adult male Australian fur seals). No cases of shark egg sheaths and rock lobsters have been found in the daily diet of New Zealand and Australian fur seals (Page et al. 2005). Although there are some differences in the species of prey identified by these three species, the sample size (20) in this study is too small to test for competitive overlap between these species in the same domain.
Recommendations for future dietary research
Further research is needed to examine seasonal dietary variations of Australian sea lions across the entire range and across different genders and age groups. Considering that Australian sea lions coexist with New Zealand fur seals and, to a lesser extent, Australian fur seals in most of their range of activity, it is important to assess how these species allocate their prey resources. Determining the caloric content of Australian sea lion prey species will help to better understand the foraging behavior of Australian sea lions and the energy contribution of prey species to sea lion diets.
The relatively small amount of hard body components used for analysis in Australian sea lion feces, ruminants and gastric contents are likely to underestimate the amount of fish eaten (Lalas 1997); therefore, molecular analysis of prey remains in the faeces will provide the most representative method for identifying prey taxa in this species. Both nuclear DNA and mitochondrial DNA can be extracted from seal feces, providing information on individual seals, prey, and gut bacteria through PCR (polymerase chain reaction) (Reed et al. 1997). Since the hard parts from prey may not be ingested or may dissolve in the intestines, analysis of prey DNA will allow for the identification of more prey taxa and will avoid inaccurate identification of prey taxa due to damaged hard parts (Symondson 2002). In order to apply this dietary analysis method, it is first necessary to determine the reference DNA sequence of the prey, which has been successfully applied to marine predators (Farrell et al. 2000; Jarman et al. 2002; Deagle et al. 2005)。
May overlap with the fisheries of the Kangaroo Island area
The study showed that the food of Australian sea lions from Seal Bay included commercial species such as the South Rock Lobster, the Silver Carp Southern School Whiting and the flat-head. The commercial value of the yellow-tailed mackerel is moderate, such as for fishing and bait fish, while the commercial value of leatherjackets is lower. The extent to which australian sea lion prey overlaps with species caught by commercial fishermen in the Kangaroo Island area is unclear and needs further research.
Sharks are a food source for Australian sea lions. Australian sea lions have reportedly plundered the shark nets of South East Scalefish and Shark Fishery and are also entangled in monofilaments of gillnets (Robinson and Dennis 1988; Shaughnessy 1999)。 Filament gillnet is the most common tangle material for Australian sea lions in Seal Bay, illustrating the frequent interaction of sea lions with this fishery (Page et al. 2004).
In summary
Ruminants and gastric specimens from australian seal bay sea lions contain hard parts consisting mainly of benthic taxa. This supports previous evidence that the species feeds primarily on shallow, benthic prey, many of which are non-migratory. As part of the Australian sea lion diet, octopuses and giant squid form the largest group of prey. Although the Australian sea lion feeds on seasonally available prey, such as the lifelong cephalopods, it also makes use of year-round available prey species such as rock lobsters and many fish. Further research is needed to analyse seasonal variations in the diets of adult and young Australian sea lions to determine the possibility of interaction with homogeneous sea lion species and commercial fisheries.
Acknowledgments
Thanks go to the staff of Seal Bay Conservation Park and the many volunteers for their assistance and support while in the field. P. D. Shaughnessy and S. Gibbs read various drafts for the manuscript and provided valuable comments. Thanks also to S. Gibbs for her assistance in the confirmation of identification of prey hard parts. This study was funded by Sea World Research and Rescue Foundation Inc., Kangaroo Island Sealink and La Trobe University. Research was conducted under permit U24630, provided by the Department for Environment and Heritage, South Australia and La Trobe University Animal Ethics Permit AEC00/42(L).
References(omit)